Eco-Friendly Management Options for Sesame Webworm (Antigastra catalaunalis Dup.)
Abstract
Background:
Sesame (Sesamum indicum L; Pedaliaceae) is an important oilseed crop that contributes significantly to the Ethiopian economy through foreign exchange but it is heavily attacked by the sesame webworm which causes 83% yield loss.
Objective:
The aim was to evaluate efficacy of aqueous extracts of seven locally available bio-pesticides (Azadirachta indica, Lantana camara, Rumex patientia, Nicotiana glauca, Ricinus communis, Phytolacca dodecandra and Tagetes minuta) along with dimethoate 40% EC (standard test) and untreated control (only tap water) against sesame webworm (Antigastra catalaunalis) insect pest, and its effects on sesame yield and yield losses under outdoor conditions.
Methods:
Field experiment was conducted at Humera agricultural research center during the 2020 harvest season. Treatments were arranged in a randomized complete block design with 3 replications and applied 3 times at 2-week intervals.
Results:
ANOVA results showed that all aqueous extracts were significantly (P<0.05) higher than the control. Uninterrupted growth of the test insect resulted in an incidence of 82.59%. However, it was decreased to 28.89% and 29.63% by spraying A. indica and R. Communis aqueous extracts. A. indica was statistically equivalent to R. communis and dimethoate 40% EC in reducing damage to leaves (8.56%), flowers (10.20%), capsules (3.34%) and seeds (29.67%) yet significantly different from controls which scored 21.8%, 20.88%, 11.51%, and 79.34%, leaf, flower, capsule, and seed damage respectively. R. communis also effectively condensed injuries on leaves (8.68%), flowers (12.64%), capsules (2.66%) and seeds (23.42%). Likewise, aqueous solutions of A. indica showed impressive yields (0.71 t ha-1) followed by R. communis (0.53 t ha-1) compared with the control (0.22 t ha-1). Yield increase over control was also higher for A. indica (222.73%) and R. communis (140.91%) compared to other treatments. The maximum (69.01%) estimated yield loss was recorded in control plots due to webworm invasion in sesame.
Conclusion:
From this result, it can be concluded that A. indica and R. communis can be excellent substitutes for synthetic insecticides to increase sesame yield under outdoor conditions.
1. INTRODUCTION
Sesame (Sesamum indicum L; Pedaliaceae) is an important annual oilseed crop that contributes significantly to the Ethiopian economy through foreign exchange earnings and as a major source of national, regional and family income for over 3 million people [1]. It is a broadleaf plant that grows up to 2 meters which is erect, branched, mostly annual plant with well-developed root system and differs in size, form/shape, growth habit, color of flowers, seed size and color and composition. It is a warm season crop and is considered drought tolerant, but needs good soil moisture for establishment and good yield. It is grown in Aden, Burma Russia, Italy, Spain, Cyprus, East and West Africa. In Ethiopia, oilseeds are the third largest crop sector after cereals and legumes in area coverage. Sesame ranks first in the oilseed sector with a total area of 654,976.74 ha, an annual production of 4,939,185.09 quintals and an average annual yield of 7.54 quintals ha-1 [2]. It is the first exporter (79%) of oilseeds and the second largest agricultural exporter (20%) after coffee in Ethiopia [3]. Tigray has the highest share (36%) of the country's sesame-producing regions [4]. Tigray's average sesame planting area is higher (25 ha) than the national average of 16 ha per household. Average sesame production per household in Tigray is 62 quintals, with production varying between 0.8 and 1500 quintals per producer [5]. Sesame also has nutritional and medicinal properties [6]. Similarly, sesame is used in confectionery, tahini, cookies, cakes, breads, soaps, cosmetics, pesticides, halva, and pharmaceuticals.
Despite its importance as a major export product of the country and its importance as a nutritional and pharmaceutical asset, its production has not increased satisfactorily due to several limitations resulting in significant yield losses before and after harvest. Sesame production and productivity are very low compared to other oilseeds [7]. This is due to limited attention to yield [8] and limited advanced studies investigating the effects of biotic and abiotic stress. However, some studies have been conducted showing significant loss of sesame yield due to biotic factors [9-11]. This oil crop was reportedly damaged by 29 insect pests [12]. Notably, the most serious pre-harvest insect pest is the sesame webworm (Antigastra catalaunalis), which causes 83% yield loss [13]. Sesame is heavily attacked by this pest during the two weeks after emergence until harvesting and threshing [14]. It feeds on delicate leaves by webbing the upper leaves and burrowing into the pods and shoots [15]. It also causes 10-70% leaves, 34-62% flowers and 10-44% pods infestation resulting in 72% yield loss [16].
Chemical pesticides are the main control options of this insect in sesame production by both commercial and smallholder farmers. However, their use has many undesirable and fatal consequences. Continued use of synthetic pesticides can lead to the development of resistance and death of beneficial organisms [17]. It is also associated with potential toxic effects on human health [18], water pollution [19]. and increased production costs [20]. Particularly, the use of certain chemical pesticides on sesame can lead to the accumulation of residues on seeds, oil and cake [21]. Thus, the changing scenario of pest control concepts has brought natural products to the fore front as effective and reliable pesticide molecules for controlling pests [22]. There are various reports that many aqueous extracts of natural products can effectively control pests and are safe for human and environmental health. Therefore, there is a need for environmentally friendly alternatives to the complex pest of sesame. In keeping with this concept, the present study was conducted to evaluate potential candidate of aqueous extracts against sesame webworm, sesame yield and its yield losses under field conditions.
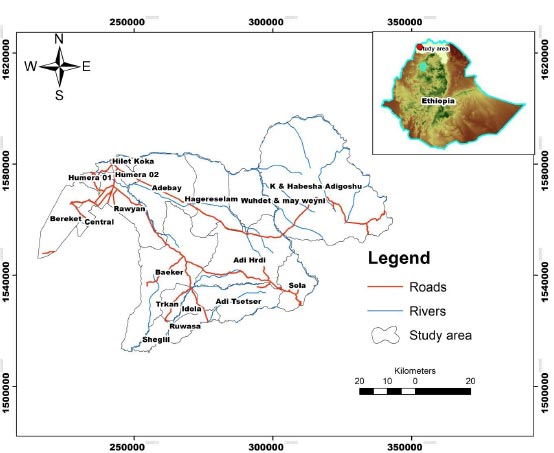
2. MATERIALS AND METHODS
2.1. Site Description
Field experiment was conducted at the Humera Agricultural Research Center during the 2020 harvest season. Humera is located in the western part of Tigray, at latitude 14°18°N and 36°37°E, longitude 14.3°E and 36.62°E. It is 600 km from Mekellle, the capital of Tigray province, bordering Eritrea to the north and Sudan to the west. It is a flat terrain with an altitude of 500 to 800m. It is characterized by arid climate conditions with an average annual temperature of 29°C and an average annual rainfall of 581.2 mm [20] (Fig. 1).
2.2. Experimental Design and Treatments
The field experiment was laid out in randomized complete block design with three replications. It was composed of nine treatments viz. seven bio-pesticides aqueous leaf extract, a synthetic chemical insecticide-dimethoate 40% EC (standard check) and untreated control (watering spray). Sesame (Setit-1 variety) was used as a taste variety because it performed better in low rainfall conditions as compared to other varieties in the study area. Seeds were sown on 20th July, 2020. Paths between plots and blocks were 1 and 1.5 m, respectively, to minimize drift during spraying. The experimental plot size was 6 m2 and contained a total of 27 plots. Each plot consisted of 5 rows and data was collected from 10 randomly selected plants in the middle 3 lines. Sesame seeds were drilled with inter-rows spacing of 40 cm. We followed other cultural practices recommended for commercial production of sesame. A detailed description of each treatment is provided in Table 1.
S.No. | Local Name | Common Name | Botanical Name |
---|---|---|---|
1 | Limo | Neem | Azadirachta indica |
2 | Alalimo | Wild Sage | Lantana camara |
3 | Shembaeta | Rumex | Rumex patientia |
4 | Chrgid | Tree Tobacco | Nicotiana glauca |
5 | Gulie | Caster bean | Ricinus communis |
6 | Shibti | Endod | Phytolacca dodecandra |
7 | Chenawi | African Marigold | Tagetes minuta |
8 | - | Dimethoate 40% EC | - |
9 | - | Control (watering spray) | - |
2.3. Extraction Methods
Mature leaves of the plant were collected from around the study area. The harvested leaves were washed and dried in the shade without direct sunlight [23, 24], finely ground into a powder using a fruit juice grinder. Powders from all treatments were filtered through a spray sieve to remove larger particles and to obtain finer powder [25]. To prepare aqueous solutions for the experiment, each powder was poured separately into prepared buckets and soaked in distilled water for 24 hours with a ratio of 50g/l [24-26]. The mixture was then repeatedly stirred with a wooden stirrer. Aqueous extraction was performed by mixing and agitating the samples [25] (Ibrahim et al., 2019). The mixture was then filtered through a muslin cloth. The solution was ready for spray application. 15 ml of soap was added for emulsification purposes [24, 26]. Soap and dimethoate 40% EC were purchased from a local market and used for subsequent field trials.
2.4. Data Collected
2.4.1. Insect Incidence and Damage Parameters
Data were collected from 10 randomly sampled plants of the middle three rows in each plot of the experiment. Spray applications were done thrice at two weeks interval. The aqueous leaf extracts were compared with respect to their efficacy against sesame webworm incidence and damaged plant parts during the study. The description, and calculation of percentages of insect incidence and damaged plant parts were done as clearly indicated below.
Incidence (INC %): the ratio of sesame webworm infested plants to the total plants in a plot was calculated using followiing equation:
![]() |
Leaf damage (LD %): leaves that have symptoms of sesame webworm attack per plant were recorded. Bored and webbed leaves were taken as damaged leaves.
![]() |
Flower damage (FD %): Damaged flowers per plant were recorded. webbed and tunneled flowers were considered as damaged flowers.
![]() |
Capsule damage (CD %): bored capsules per plant were considered as damaged capsules
![]() |
Seed loss (SL %): the ratio of seeds per each damaged capsule to the seeds per each healthy capsule was calculated using the following equation.
![]() |
2.4.2. Yield and Yield related Parameters
Plant height: Measured in cm from the soil surface to the tip of the plant at 50% physiological maturity.
Number of capsule plant-1: Counts were made at maturity from sampled plants.
Number of seeds capsule-1: Counted at maturity from sampled plants.
Hundred seed weight: Harvested grain in each plot was weighed using a sensitive balance.
Biomass yield: Determined by harvesting, drying (outdoors) and weighing the middle row of plots. Grain yield: Plants harvested from the middle row of plots were manually threshed, cleaned, sun-dried, weighed and determined after adjusting the moisture content to 12.5% with a grain moisture meter.
Avoidable yield loss: computed from the grain yield recorded in untreated plots and those receiving maximum protection against sesame webworm. It was computed by:
![]() |
Where: C = avoidable loss (%); a = grain yield in protected plots, and b = grain yield in unprotected plots.
2.5. Data Analysis
The generated data were analyzed using SAS software version 9.00 [27]. Treatment means were compared using the least significant difference (LSD) test at the 5% level using Fisher's multiple comparison tests.
3. RESULTS AND DISCUSSION
3.1. Insect Incidence and Sesame Damages Affected by different Aqueous Extracts
3.1.1. Incidence of Sesame Webworm (%)
Sesame webworm incidence was found to be significantly lower (p<0.01) in all treatments compared to controls (Table 2). All aqueous extracts showed bio-pesticidal activity by reducing sesame webworms to significant levels. Among the aqueous extracts, A. indica leaf extract had the lowest (28.89%) prevalence, followed by R. communis leaf extract (29.63%) both of which were statistically similar with dimethoate 40% EC (standard test). The highest (82.59%) sesame webworms incidence was recorded from the controls which was significantly higher than the treated plots (Table 2). This result is consistent with those who reported a significant reduction in webworm infestation using A. indica extract [28]. A. indica leaf and seed kernel extracts at 30 ml/l are highly effective in controlling larval populations of the sesame webworm [29]. Therefore, 15% A. indica water extract performed well over two seasons, resulting in 100% mortality under laboratory conditions [28]. Higher mortality of P. brassicae (19.6% and 18.5%) was observed in M. azedarach and A. indica aqueous extracts [30]. Mortality of tomato leaf miners ranged from 33% to 46.7% in 24 hours, reaching 100% mortality 4 days after application of A. indica extract [30]. It is also reported that an aqueous extract of R. communis was toxic to P. brassicae, resulting in 8.9% mortality on cabbage [31]..Similarly, more than 80% mortality of whiteflies and 70% of scale insects were reduced by A. indica leaf extract [25]. [24] Investigated 80% and 63% mortality of tomato leaf miner by spraying R. communis oil and A. indica leaf extracts. Azadirachtin, a compound derived from the neem tree, caused insecticidal activity by interfering with hormones that control insect molting [32]. A neem-based formulation was found to be effective in reducing sesame pests by 5%, and nimbecidin was one of the commercial formulations tested to be effective against sesame leaf webbers [29]. It has also been reported a repellent effect of an aqueous extract of M. azedarach and A. indica against larvae of P. xylostella [33]. The antifeedant activity of lantana extract was demonstrated by [34] who reported that complete inhibition of cabbage leaves treated with 1% L. camara against P. brassicae. Similarly [35], reported that an aqueous extract of L. camara reduced the invasion of Helopeltis theivora from 38.90% to 27.63% on tea leaves. Based on the results obtained and discussed above, A. indica and R. communis were highly effective against sesame webworms (Table 2).
3.1.2. Leaf Damage (%)
Statistical analysis shows that all aqueous extracts significantly reduced leaf damage compared to controls (Table 2). The lowest mean percentage of leaf damage was recorded in plots treated with A. indica (8.56%) and R. communis (8.68%) compared to controls (21.8%). This result is consistent with those who reported that spraying A. indica leaf extract at 30 ml/l condensed the percentage of leaf, flower and capsule damage [24, 36]. Also reported that A. indica and R. communis were as effective as dimethoate 40% EC in reducing tomato leaf and fruit by tomato leaf miners. Application of A Indica leaf and seed kernel extracts were effectively controlled sesame webber populations with two sprays, pooled over a period of time, and the sprays also affected sesame leaf, flower and capsule damage [37]. Similarly [38], reported 100% protection to cabbage leaves with petroleum ether extract at 1% of A. indica, R. communis and L. camara against Henosepilachna vigintioctopunctata.
Treatments | INC (%) | LD (%) | FD (%) | CD (%) | SL (%) |
---|---|---|---|---|---|
Azadirachta indica | 28.89d | 8.56b | 10.20b | 3.34bc | 29.67bc |
Lantana camara | 45.18bcd | 11.74b | 14.28b | 3.29bc | 35.24bc |
Rumex patientia | 39.26bcd | 13.19b | 15.30bc | 3.12bc | 37.47bc |
Nicotiana glauca | 54.44b | 12.30b | 13.32b | 3.20bc | 49.77 b |
Ricinus communis | 29.63cd | 8.68b | 12.64b | 2.66bc | 23.42bc |
Phytolacca dodecandra | 45.18bcd | 10.97b | 15.32bc | 3.18bc | 32.39bc |
Tagetes minuta | 48.88bc | 13.39b | 17.64bc | 4.93b | 44.93bc |
Dimethoate 40% EC | 28.15d | 9.29b | 11.32b | 2.89bc | 18.49c |
Control | 82.59a | 21.80a | 20.88a | 11.51a | 79.34a |
CV % | 25.84 | 38.00 | 32.90 | 42.11 | 41.67 |
LSD (0.05) | 19.99 | 8.03 | 8.46 | 3.00 | 28.11 |
3.1.3. Flower Damage (%)
Floral damage was significantly (p<0.05) affected by the application of the aqueous extract over the control (Table 2). Comparing all extracts, plots treated with A. indica (10.2%) followed by R. communis (12.64%) scored a lower percentage of flower damage, whereas the control showed a higher percentage (20.88%) of flower damages. Similar results were reported by [37] who found that the application of 5% A. indica leaf and seed extract reduced flower damage by 14.41% and 12.92%, respectively. R. communis (12.64%), N. glauca (13.32) and L. camara (14.28) were significantly comparable to dimethoate 40% EC (standard test) but significantly superior to the control. In addition, R. patientia, P. dodecandra and T. minuta was not significantly different between them and with dimethoate 40% EC, but was still higher than the untreated controls.
3.1.4. Capsule Damage (%)
ANOVA results showed that all plots treated with aqueous extracts showed the lowest capsule damage compared to controls (Table 2). Among the extracts, minimal capsule damage (2.66%) was recorded by R. communis, which was statistically at par with A. indica, L. camara, R. patientia, N. glauca and P. dodecandra aqueous extract treated plots except T. minuta (4.93%). The extent of capsule damage caused by T. minuta is twice as large as R. communis, indicating superior damage reduction potential compared to controls (11.51%). The results are consistent with [37] who reported that spraying an aqueous extract of 5% A. indica seed extract was (10.78%) better in reducing capsule damage than the untreated (24.61%) control [39]. also reported that the use of A. indica aqueous extract reduced capsule damage to 7.75% compared to 11.44% in controls. Minimal (2.03–2.43%) capsule damage was also reported by spraying A. indica seed extract in combination with other IPM sequences [40]. Similarly [41], reported that 19.72% to 21.62% damage to sesame pods was evident when unprotected and decreased from 3.02% to 3.12% damage when protected.
3.1.5. Seed Loss (%)
Statistical analysis shows that all treated plots were found to reduce seed loss more than untreated controls (Table 2). Plots sprayed with R. communis (23.42%) followed by A. indica (29.67) observed the lowest percentage of seed loss per damaged capsule, but the highest percentage (79.34%) of seed loss per damaged capsule was recorded in the control group. All aqueous extracts significantly reduced seed losses compared to the control but they didn’t show significant variations among themselves except N. glauca which scored 49.77% of seed loss per the broken capsule. This result was in agreement with the findings of [28] who found that an aqueous extract of A. indica at 15% prevented feeding of all larvae tested, while the larvae treated with 10% A. indica having a 2.2 mg/larva (1.8%) compared to untreated control 3.5 mg/larva (31.4%) and starvation control seed loss was 0.62 mg/larva (33.2%). In the current study, we observed statistically equivalent seed loss by sesame webworms with the application of A. indica and R. communis, but far superior to controls (Table 2).
3.2. Yield and Yield related Traits influenced by different Aqueous Extracts
3.2.1. Plant Height
A significant variation (P < 0.05) in plant height was absorbed when the aqueous extract was tested (Table 3). The highest plant height (113.22) was recorded from the R. communis treated plot and the shortest plant height (91.28) was obtained from the untreated control. This result indicated that sesame webworm infestation had a significant effect on plant height, by causing plant growth inhibition. The rest of the aqueous extract was significantly comparable to the synthetic insecticide (dimethoate 40 EC) used as a standard test in this study (Table 3).
Treatments | PH (cm) | NCPP | NSPC | Biomass Yield (t ha-1) |
Hundred Seed Weight (g) |
Grain Yield (t ha-1) | Yield over Control (%) | Avoidable Yield Loss (%) |
---|---|---|---|---|---|---|---|---|
Azadirachta indica | 101.78ab | 29.48ab | 112.56a | 3.09ab | 0.46a | 0.71a | 222.73 | 69.01 |
Lantana camara | 94.17ab | 18.39ab | 100.44ab | 2.28bc | 0.33bc | 0.35bc | 59.10 | 37.14 |
Rumex patientia | 98.00ab | 23.61ab | 89.89ab | 2.77ab | 0.36ab | 0.45b | 104.55 | 51.11 |
Nicotiana glauca | 102.28ab | 29.44ab | 95.00ab | 2.28bc | 0.36ab | 0.42b | 90.91 | 47.62 |
Ricinus communis | 113.22a | 29.38ab | 103.33ab | 3.03ab | 0.39ab | 0.53ab | 140.91 | 58.50 |
Phytolacca dodecandra | 91.50b | 23.11ab | 93.56ab | 2.34bc | 0.26bc | 0.38bc | 72.73 | 42.12 |
Tagetes minuta | 103.56ab | 26.50ab | 99.11ab | 2.79ab | 0.36ab | 0.44b | 100.00 | 50.00 |
Dimethoate 40% EC | 102.22ab | 35.33a | 103.89ab | 3.24a | 0.45a | 0.70a | 218.18 | 68.57 |
Control | 91.28b | 17.61b | 83.22b | 1.73c | 0.25c | 0.22c | 0.00 | 0.00 |
CV % | 12.46 | 38.24 | 16.63 | 18.80 | 17.28 | 23.27 | - | |
LSD (0.05) | 21.65 | 17.12 | 28.18 | 0.85 | 0.11 | 0.19 |
3.2.2. Number of Capsule per Plant
ANOVA shows a significant (P < 0.05) difference in the number of capsules per plant (Table 3). The highest (35.33) number of capsules per plant was recorded by a standard test (dimethoate 40% EC). Correspondingly, the highest number of capsules per plant was recorded from aqueous leaf extracts of A. indica (29.48), N. glauca (29.44) and R. communis (29.38), each of which was significantly comparable, but the lowest number of capsules per plant (17.61) was observed in the untreated control (Table 3). This result indicates that the high number of pods may be due to the presence of healthy plants (leaves and flowers) capable of producing a large number of fertile pods.
3.2.3. Number of Seeds per Capsule
Statistical analysis shows that all aqueous extracts significantly (P < 0.05) increased the number of seeds per capsule over the control (Table 3). Aqueous leaf extracts were examined and the highest number of seeds per capsule (112.56) was obtained from A. indica, followed by R. communis leaf extract, yielding 103.33 seeds per capsule, each of which was significantly equivalent with dimethoate 40% EC (standard test). However, the lowest number of seeds per capsule (83.22) was found in the control. This result confirmed that the lower number of seeds per capsule in the control plots was probably due to a higher percentage of damaged plant parts (leaves, flowers, capsules). This limits the production of healthy capsules and reduces the number of seeds.
3.2.4. Hundred Seed Weight
A significant difference (P < 0.05) was observed between the aqueous extract and the control on hundred seed weight, except for L. camara, which was not significantly different from the control (Table 3). The highest seed weight (0.46 g) was obtained from A. indica leaf extract, but was significantly comparable to the standard test dimethoate 40% EC while the lowest (0.25 g) seed weight was recorded from the control plot. There was no significant difference between R. patientia, N. glauca, R. communis and T. minuta extracts on the seed weight, however, all of which were significantly varied from the untreated controls.
3.2.5. Biomass Yield
Application of different aqueous extracts showed significant (P < 0.05) variation in biomass yield (Table 3). Among the extracts, higher biomass yields were observed from A. indica (3.09 t ha-1), followed by R. communis (3.03 t ha-1), T. minuta (2.79 t ha-1) and R. patientia (2.77 t ha-1) compared with controls (1.73 t ha-1). All were statistically equivalent to dimethoate 40% EC (standard test). Similarly, aqueous extracts of A. indica and M. azedarach repelled the highest number of P. brassicae, protecting 94% and 89.2% of cabbage foliage’s [30]. They also found that aqueous extracts of A. indica (81.8%) and M. azedarach (81.7%) provided statistically the same protection against cabbage leaves at 10%, however maximum protection was provided at 5% of M. azedarach (88.3%) and A. indica (82.5%) against P. brassicae. The remaining extracts did not provide better biomass yield than the control. In other expression, L. camara, P. dodecandra, and N. glauca produced biomass yields that were statistically similar to controls.
3.2.6. Grain Yield
ANOVA showed a significant (P < 0.05) difference in grain yield (Table 3). The highest grain yields (0.71 t ha-1) were harvested from plots treated with A. indica followed by R. communis (0.53 t ha-1), and statistically at par with dimethoate 40% EC the standard test. However, the lowest grain yield (0.22 t ha-1) was harvested from the untreated control plot. All the aqueous extracts showed no significant variation in grain yield among themselves, except for A. indica which produced the highest grain yield. This finding was consistent with the results of [37] reported that higher seed yields (529.80 kg ha-1) and (495.66 kg ha-1) were harvested in plots sown with 5% A. indica seed extract in the summer and kharif seasons, respectively. Maximum seed yield (8.21 q ha-1) was also recorded by spraying A. indica seed kernel extract in combination with other IPM techniques and sequences [40]. [41] stated that the highest (921.42 kg ha-1) sesame yield was recorded under protected conditions and the lowest (521.48 kg ha-1) yield was harvested under unprotected conditions [42]. Who reported that the highest sesame yield (714 kg ha-1) was recorded in plots treated with dimethoate 40% EC applied at 2 l ha-1 to sesame webworms. In this study, aqueous extracts of A. indica and R. communis were unexpectedly found to protect both biomass and grain yield over the control against sesame webworms (Table 3).
3.2.7. Avoidable Yield Loss
The avoidable yield loss and yield gain over the control is presented in Table 3. The highest (0.71 t ha-1) grain yield was harvested from A. indica treated plot and the lowest (0.22 t ha-1) grain yield was harvested from the untreated control plot. This indicates that the greatest avoidable yield loss was from A. indica (69.01%) and R. communis (58.50) treated plots compared with other aqueous extracts due to sesame webworms. In contrast, the untreated control plot recorded a maximum estimated yield loss of 69.01% due to the insect infestation. In general, a yield advantage of up to 222.73% was obtained from plots treated with A. indica leaf extract, whereas overall yield increases with the different aqueous extracts used in the experiments ranged from 59% to 222.73%. This finding was consistent with [28] where 53% increase in sesame yield in plots treated with aqueous extracts of A. indica compared to untreated controls was reported. The avoidable yield loss of sesame due to webworms was found to be up to 39.73 after three times application of bio-pesticide [41]. In the same way [43], was reported a maximum of (57.60%) avoidable yield loss caused by insect pests in sesame.
CONCLUSION
Among the seven aqueous extracts evaluated their efficacy against sesame webworms (Antigastra catalaunalis Dup), Azadirachta indica and Ricinus communis were found to be effective in controlling webworms development and sesame damage densification. However, the rest were not very effective at controlling the insect pest and recorded low mortality. This indicates that A. indica and R. communis extracts may possess antifeeding properties and toxicants that can help effectively act against the target insect. The insect infestation coincided with the growth of new flushed leaves, flowers and capsules, thus A. indica and R. communis should be matched to these sensitive parts and harvest stages to minimize damages of the crop. A. indica and R. communis also produced higher biomass and grain yields with higher avoidable seed yield losses. The maximum estimated yield losses of 69.01% and 58.50% were protected from webworms infestation by application of A. indica and R. communis aqueous extracts respectively. From this result, we can conclude that A. indica followed by R. communis aqueous leaf extracts were found to be as effective as a synthetic insecticide (dimethoate 40% EC) and, even better in reducing the target insect pest and saving sesame yields. The above mentioned aqueous extracts are locally available, inexpensive, and environmentally friendly, so it may be possible to recommend and encourage growers to use them as part of an integrated management strategy to control sesame webworms.
AUTHORS’ CONTRIBUTION
Alemu Araya Kidanu: Apprehended, designed and performed the experiments; prepared the aqueous extracts, interpreted the data and wrote the manuscript. Tarekegn Yohannes Wube: Perceived and designed the experiment, analyzed the data and wrote the paper. Ibrahim Fitiwy Beyan: Performed the experiment. Abraha Gebretsadkan Berhe: Prepared extracts and reagents and executed the experiment.
LIST OF ABBREVIATIONS
LD | = Leaf damage |
FD | = Flower damage |
CD | = Capsule damage |
SL | = Seed loss |
CONSENT FOR PUBLICATION
Not applicable.
AVAILABILITY OF DATA AND MATERIALS
All the source of data and materials used were our bonafide purpose and available upon the request of the corresponding author [A.A].
FUNDING
This study was funded by Mekelle University, (Funder ID: MU; Recurrent Budget).
CONFLICT OF INTEREST
The authors declare no conflict of interest financial or otherwise.
ACKNOWLEDGEMENTS
We sincerely thank Mekelle University for their financial support during this study. We are also particularly grateful to the Department of Dryland Crop and Horticulture crop protection laboratory, which has access to drying, crushing and extraction of harvested plants.