All published articles of this journal are available on ScienceDirect.
The Technology Used for Synthetic Polyploid Production of Miscanthus as Cellulosic Biofuel Feedstock
Abstract
Background:
The contemporary bioethanol production technologies are based on the utilization of plant lignocellulosic biomass. These technologies require conducting regular search, breeding, and creation of new energy crops. In particular, significant attention is paid to plants of the genus Miscanthus - perennial grasses that have a great potential as renewable energy sources. The main advantages of representatives of this species are considered to be high biomass yield, cold tolerance, a low requirement to soil conditions, long-term use of plantation, etc. M. × giganteus is the most promising species of the genus. The last is a sterile allotriploid originated through the hybridization of M. sinensis and M. sacchariflorus. Due to the problem with sexual reproduction, there is a lack of genotypes necessary for plant breeding programs to improve this species. Thus, polyploidization is an indispensable approach for obtaining new genotypes of M. × giganteus.
Objective:
The aim of this work is to review the attempts and methodologies employed to induce polyploidy in plants belonging to the genus Miscanthus.
Methods:
For this purpose, the concentrations and duration of treatment with different antimitotic agents in species, within this genus, have been considered. Methods for ploidy level determination and evaluation of biological and biochemical traits in the resulting polyploids have also been reported.
Results:
The application of antimitotic agents in vitro is the most effective and commonly used method of polyploidization in Miscanthus. The most effective antimitotic compounds appeared to be the well-known dinitroanilines, such as oryzalin and trifluralin, as well as new dinitroanilines with significantly lower phytotoxicity level.
Conclusion:
Polyploidization in Miscanthus has been investigated by various research groups worldwide. Currently, polyploid forms of M. sinensis, M. sacchariflorus and M. × giganteus have been obtained. The biological and biochemical traits of the obtained polyploids differ significantly from their original forms. However, the challenge of fertility restoration of M. × giganteus has not been resolved yet.
1. INTRODUCTION
Biomass has the greatest perspectives among different renewable energy sources. Biomass is an organic compound of vegetable or animal origin that is used as an alternative energy source. Nowadays, approximately 10% of the energy used worldwide is produced from biomass. The percentage of biomass in energy balance grows steadily, year by year. The
percentage of biomass in the EU countries for 2020 is expected to be >10% of all energy types used in the transport sector [1]. The main sources of biomass are of vegetable origin: cereals straw, husk, woody wastes, and energy crops, which are solely grown for energy production [2].
Miscanthus is a representative of perennial grasses. It gained popularity as an energy crop in regions with temperate climate because of its’ many positive features, among which are high biomass yield, adaptation to low fertility soil, cold tolerance, and C4 photosynthesis [3]. Miscanthus shows higher rates of leaf photosynthesis than switchgrass owing to its higher water, nitrogen, and light use efficiencies, and as a result, it has increased biomass productivity [4]. Under optimal growing conditions, the yield of M. × giganteus biomass reaches up to 39.89 t/ha of dry mass. These data are also of importance in the light that biomass harvesting of commercial plantations of M. × giganteus lasts up to 20 years [5].
Representatives of the genus Miscanthus are disease resistant, and thus, they do not require any extra chemical protection. The fertilizers requirement for the species is also very low. With normal fertilization and average dry matter productivity of 20 t/ha, Miscanthus takes out from the soil approximately 60 kg of N, 16 kg of P2O5, and 80 kg of K2O. Moreover, M. × giganteus plantations sequestrate carbon into solid organic form in the soil, therefore preventing greenhouse gas accumulation in the air and global warming, which nowadays, are among the greatest problems [6]. The net soil carbon sequestration of M.× giganteus plantation is 0.64 t C ha-1 y-1, which is twice higher than the net soil carbon sequestration of willow plantation (0.30 t C ha-1 y 1) [7].
Among other species of this genus, M. × giganteus has the highest content of cellulose, approximately 68-70%. Thus, this species is a valuable raw material for the paper industry and is also utilized in building materials production industry. Miscanthus is the prior bioenergy crop for advanced and 2-nd generation technologies of biofuel production [8].
M. × giganteus is allotriploid resulting from the union of the unreduced gamete of M. sacchariflorus and gamete of M. sinensis. It is known that genome sizes of M. × giganteus, M. sinensis and M. sacchariflorus are 7, 5.5 and 4.5 pg, respectively [9]. As in the vast majority of interploidy hybridization cases, M. × giganteus has significant problems with its fertility, including pollen incompatibility. The creation of triploid forms also requires overcoming the phenomenon of the triploid block, which leads to the formation of non-viable seeds [10, 11]. On one hand, the sterility of M. × giganteus gives an advantage to this crop owing to risk absence of its spreading over the field borders into the natural environment with seeds or through sexual hybridization with wild cereals by cross pollination process [12]. However, on the other hand, it makes the selection process of M. × giganteus impossible.
Breeding in Miscanthus genus is based on repeated recombination cycles that follow the crossing between genetically different genotypes in order to accumulate positive alleles and reduce those for undesirable traits [13]. The breeding process takes a long time, and for representatives of Poaceae family, the typical breeding cycle takes about 7 years [14]. Therefore, problems with sexual hybridization of M. × giganteus lead to a reduction of useful genetic diversity that is essential for the breeding of this species. All commercial M. × giganteus plantations comprise only one vegetatively propagated genotype. It is very important to obtain genetic variations of M. × giganteus with restored fertility for involving them into breeding processes with the aim of improving productivity and quality of raw material, resistance to pests and diseases [15, 16]. Thereby, in the case of sterile M. × giganteus, polyploidization is an indispensable method for obtaining new genotypes. Recently, a successful study on polyploidy induction with dinitroanilines has resulted in fertility restoration of intergeneric hybrid between Chitalpa and Chilopsis [17]. Fertile polyploids of Napier grass (Pennisetum purpureum) have been obtained with the application of dinitroanilines [18]. Thus, the application of polyploidy in plant breeding has resulted in the development of new, increasingly productive, and well-adapted cultivars [19]. New highly productive hybrids based on heterosis phenomenon can also be obtained by using the new polyploid genotypes of Miscanthus. Moreover, polyploidization provides the opportunity for hybridization among hexaploid fertile genotypes of M. × giganteus or interspecific crossings among tetraploids of M. sinensis, M. sacchariflorus, and others [20]. Obtaining polyploid lines is important due to the advantages of polyploids over their original forms, such as larger cell size and higher biomass productivity [21].
There are two mechanisms of polyploidy induction: mitotic polyploidization when chromosome number of somatic tissue is doubled and the generation of 2n gametes in meiotic polyploidization [22]. Doubled haploids can be obtained by two methods: using anther culture in order to change the microspore development pathway and gynogenesis. Both methods are effectively used and have both advantages and disadvantages [23-27]. Protocols for in vitro polyploidization have been developed and successfully applied since 1966. The main reason is that tissue culture methods allow micropropagation of plant material at a significantly larger scale compared with propagation in the greenhouse and, moreover, the former ensures standardized and controlled conditions of treatment [10]. Chromosome doubling for production of tetraploids, fertile amphidiploids, and hexaploids in the genus Miscanthus [20, 28-30] as well as in other related species [31], using compounds with antimitotic activity, has been successfully applied. The most common compounds with antimitotic activity are colchicine, oryzalin, and trifluralin. They bind tubulin dimers during cell division, and by doing so, they prevent the formation of microtubules and spindle fiber organization [29]. Colchicine is the most popular drug used for chromosome doubling since 1930 [32]. However, in many species, colchicine treatment leads to sterility, chromosome losses and rearrangement, abnormal growth, and gene mutation [33]. Thus, since 1990, the attention of scientists has been concentrated on the use of other compounds instead of colchicine.
Phosphorothioamides is a class of compounds with antimicrotubular activity that have been used as an alternative to colchicine [34-39]. Oryzalin and trifluralin are dinitroanilines which gained popularity as pre-emergence herbicides. Their mode of action lies in the depolymerization of microtubules and thus, in preventing the development of a plant root system [40]. Despite the similarity in the mode of action of all these compounds and the highly conserved nature of the tubulin sequences, the affinity of colchicine and its related compounds is very low for plant tubulin. Therefore, dinitroanilines had become widespread as compounds capable of plant microtubules depolymerization more efficiently compare with colchicine [41]. One more important aspect is that colchicine, due to its high affinity to tubulins of animal origin, provides very tight linkage among them, thus is very toxic for humans [42]. At the same time, dinitroanilines have a low affinity to fungal and animal tubulins but show a very high affinity to plant tubulins [43-46].
However, dinitroanilines affect plant cells not only for blocking polymerization of mitotic microtubules that become unable to form spindle fiber, but also in destroying microtubules of the cell cortex during interphase. This causes proliferative death of sensitive cells [39]. Thus, the application of classic dinitroanilines for polyploidization has problems owing to their high phytotoxicity. Several studies on plant polyploidization highlighted problems with high phytotoxicity of oryzalin and trifluralin to dicotyledonous [34] as well as to monocotyledonous plants [47], including Miscanthus [20]. Similar results have been obtained in our laboratory using oryzalin and trifluralin in polyploidyzation of M. × giganteus and M. sinensis. In general, according to Dhooghe et al. (2011), the optimal concentrations of the antimitotic agent and exposition time are highly species-dependent. Today, colchicine is still often used to induce polyploidy of fruits, vegetables, and agricultural crops. However, oryzalin and trifluralin are most common in polyploidization in ornamental plants [48].
There are other perspective dinitroaniline compounds for polyploidy induction that may be used successfully, these include N,N-diethyl-2,6-dinitro-4-(trifluoromethyl)aniline and N-(2,6-dinitro-4-trifluoromethylphenyl)propanol. Processes of tubulin polymerization are significantly affected or completely blocked after the application of these compounds as a result of their interaction with plant tubulin [49, 50]. Previously, the spatial structure of α-tubulin molecules from Miscanthus was reconstructed and binding site of a dinitroaniline was verified in silico methods, as shown in Fig. (1) [51].
The ability of new and well-known compounds of dinitroanilines to form a ligand-protein complex with miscanthus α-tubulin (Q70ZL7) has been evaluated as well. The most promising compounds for polyploidization of Miscanthus genus have been selected and described earlier [51].
2. MISCANTHUS POLYPLOIDIZATION WITH COLCHICINE
A number of studies on in vitro polyploidization of different representatives of Miscanthus genus, using colchicine, have been conducted [20, 28, 29, 52]. Petersen et al. (2003) investigated parameters, such as colchicine concentration, exposition, and type of explants, for the polyploidization of M. sinensis. Plants in vivo and shoots in vitro, shoot apexes, leaf explants, as well as in vitro stems and embryogenic calli induced from immature inflorescences have been used as explants for antimitotic treatment. After the treatment of M. sinensis plants with colchicine, it was found that the number of new shoots in 12 weeks post-treatment (PT) has decreased with increasing of colchicine concentration. Thus, when plants were treated with 626 µM colchicine, the number of new shoots was 52, however, after treatment with 1252 and 2404 µM, the shoots formed after 12 weeks were only 30 and 14, respectively. These results are consistent with previous studies on the treatment of M. sinensis plants with colchicine [29]. In this experiment, plants from soil have been washed with water and treated with colchicine diluted in water. In order to enhance the absorption of an antimitotic substance by tissues, the solution contained 1.5% dimethyl sulfoxide (DMSO). After colchicine treatment, the plants were cut and 5 cm long shoots were planted in the greenhouse. At 12 weeks PT, the number of surviving plants and number of newly formed shoots were counted and ploidy level of separate shoots was determined by flow cytometry. As a result, no tetraploid plants of M. sinensis were obtained and only 3 shoots out of 52, obtained after colchicine treatment at a concentration of 626 µM, had both tetraploid and diploid nuclei, and only 1 shoot out of 14. obtained after colchicine treatment at a concentration of 2404 µM, was chimeric [28].
After the colchicine treatment of in vitro grown shoots of M. sinensis with 156, 313, 626, 1252, and 2504 µM for 6 and 18 h, viable shoots were found at concentration lower than 313 µM. In this study, the best results were observed with 156 μM colchicine treatment for 6 h, since the number of obtained tetraploid plants was the highest - 39.3%. Moreover, 99 plants were obtained from 6 treated shoots after 12 weeks of cultivation. Subsequently, 84 of those were tested and 33 of them were found to be tetraploids. From 6 shoots treated with 313 µM colchicine for 18 h, 27 new shoots were obtained after 12 weeks, among which, 2 were tetraploid and 2 chimeric. Because only a few tetraploid and a large number of chimeric plants have been obtained after treatment of other M. sinensis genotypes, it has been argued that colchicine treatment is genotype-dependent [29].
In vitro plantlets of M. sinensis have been treated with colchicine as well. After rooting on the root induction medium, plants were immersed for 6 h in colchicine solution at concentrations of 156 and 313 µM. The treatment was carried out under light and at a temperature of 24 °C. After treatment, plants were cut, and 5 cm long shoots were acclimated and grown in a greenhouse. The survival rate of explants has been estimated to be over 4 weeks after treatment. The number of obtained shoots and their ploidy level was determined 9 weeks after treatment. According to this study, 7 genotypes of M. sinensis did not show significant difference in the survival of explants after 4 weeks from colchicine treatment at 156 and 313 µM, however, a significant difference in a number of shoots obtained from one treated explant in 9 weeks after such treatment was observed. Thus, the treatment of 64 plants with 156 μM colchicine resulted in 395 shoots, among them, 9 were found to be tetraploid and 15 chimeric. After the treatment of in vitro plantlets with colchicine at a concentration of 156 μM, the number of new shoots regenerated per treated shoot was 6.2. Treatment with 313 µM colchicine resulted in about 4.5 new shoots regenerated per treated shoot . Thus, out of 62 treated plants, 279 shoots have been obtained, 7 of which were tetraploid and 22 had both diploid and tetraploid cells [29].
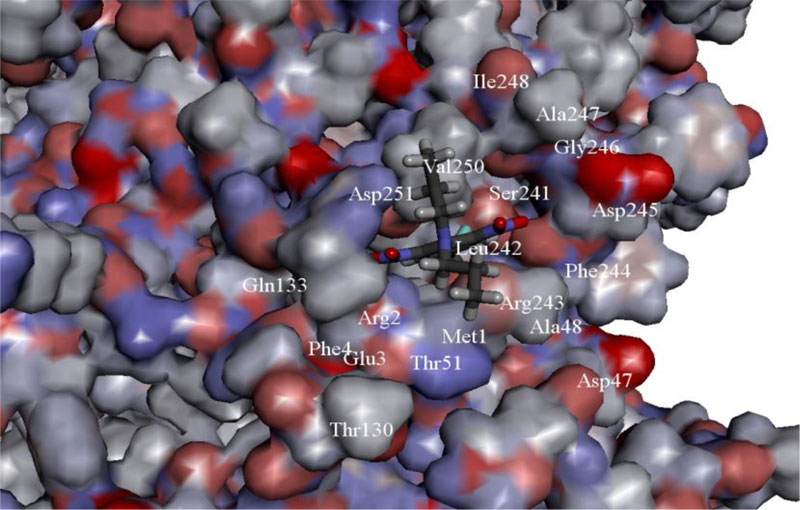
The effects of colchicine on callus induction from immature inflorescences, shoot apexes, and leaf fragments have been investigated as well. It has been found that treatment with colchicine during callus induction is more effective than plant treatment since most of the regenerated M. sinensis plants appeared to be tetraploids. The most suitable type of explant is shoot apices because the induction of shoots from callus of shoot tips is less genotype-dependent than from embryogenic calli. Best results have been obtained on medium for callusogenesis induction in the presence of 2.5 g/l colchicine for 4 days. As a result, about 80% of the treated apexes regenerated tetraploid plants [29].
Another group of researchers also conducted studies on colchicine polyploidization of M. sinensis and M. × giganteus. The efficiency of colchicine treatment of in vitro shoots [52], as well as the efficiency of colchicine treatment during induction of callusogenesis and during plant regeneration from callus has been studied [30]. Treatment of grown shoots with colchicine has been conducted on 5 cm in length shoots for 6, 8, and 24 h at different concentrations- 156.5, 313, 626, and 1252 µM. After treatment, shoots were washed three times with sterile water and transferred on medium for micropropagation MS [53] containing 2 mg/l BAP (6-benzylaminopurine). Plants were cultured for 12 weeks. After the 12 weeks cultivation, 524 explants survived out of 1800 treated, thus the percentage of the survival rate of the explants was 29.1%. No explants of M. sinensis or M. × giganteus survived at colchicine concentration of 1252 µM for 24 h. Under this condition, two other genotypes, MS11 and MS16, showed survival rates of 3.3 and 10%, respectively. However, as a result of this treatment, no polyploid lines have been obtained. The most efficient treatment for M. sinensis polyploidization was with 313 µM colchicine for 18 h. Under such condition, the percentage of polyploids was as high as 55% and the survival rate of explants was 20%. As long as M. × giganteus is concerned, the most efficient treatment of shoots was at a concentration of 1252 µM colchicine for 18 h, which resulted in polyploidization and survival rates of 7.1 and 23.3%, respectively. Under the treatment with 626 µM colchicine for 6 h, the efficiency of polyploidization was 4.3% with the explants survival rate of 33.3%, while these parameters at the same concentration and 18 h long treatment were 4.1% and 36.7%, respectively. The decrease in colchicine concentration to 313 µM led to an increase in the survival rate of explants (53.3% of the explants survived even after treatment for 24 h) with an efficiency of polyploidization of about 3.6%. No other conditions lead to polyploidization in M. × giganteus shoots [52].
A study on polyploidization of M. × giganteus and M. Sinensis during induction of callusogenesis with colchicine at concentrations of 313 and 626 µM has been conducted [30]. Immature inflorescences cultured on medium for callus induction supplemented with colchicine for the first 2, 4, or 7 days were used as explants for callusogenesis. After 12 weeks of cultivation 30238 pieces of calli were obtained from 5040 explants and then regenerated. The ploidy level of the resulting plantlets was determined and 313 µM for 7 days appeared to be the most efficient condition for polyploidization in M. sinensis. Out of the 207 analyzed shoots, 60 were polyploids, corresponding to a polyploidization rate of 29%. In most of the cases, the increase of colchicine concentration to 626 µM did not yield M. sinensis polypdoid lines. In M. × giganteus, the most efficient treatment in callus induction medium was with 626 µM colchicine for 4 days when 29 hexaploid lines had been obtained from 101 analyzed shoots, with an efficiency of polyploidization as high as 28.7%. However, under this colchicine concentration, the extension of the treatment to 7 days resulted in no polyploids. It is noteworthy that 2 genotypes of M. sinensis (MS16, MS21) and a genotype of M. × giganteus (MG1) used in the study had a higher frequency of callusogenesis induction on medium containing colchicine than in colchicine-free medium (control).
The efficiency of colchicine treatment during plantlets regeneration from calli has been studied. For these purposes, calli were cultured for the first 2, 4, or 7 days in presence of 313 µM colchicine in the regeneration medium. As a control, calli were directly transferred from callus proliferation medium to regeneration medium that did not contain colchicine. After twenty weeks, the callus cultivation on the regeneration medium shoots were been transferred onto a rooting medium and ploidy level of the obtained plants were determined. In M. Sinensis, the ability to regenerate plants from callus was found to be genotype-dependent. The study resulted in 4716 shoots of both miscanthus species. Callus pre-cultivation on colchicine-free regeneration medium for 4 days under light conditions before colchicine treatment was found to be the most efficient for M. × giganteus and all genotypes of M. sinensis. Thus, out of the 136 M. × giganteus plants regenerated on the medium supplemented with colchicine for 4 days, 28 or 20.6% of the plants were found to be hexaploids. In such case, the highest polyploidization efficiency (40%) in M. sinensis was obtained for the genotype MS19. Despite that, only 10 out of the 25 plants, regenerated under such conditions, were hexaploid. Once again, these results suggest that successful polyploidization by colchicine in this species is genotype-dependent. For diploid M. sinensis genotypes, the most efficient condition was when calli had been cultivated on medium with 313 µM colchicine for 2 days without callus pre-cultivation under light conditions, since 69 or 26.9% out of the 233 obtained plants were regenerated tetraploids [30].
In another laboratory, calli induced from immature inflorescences of M. × giganteus have been used as material for polyploidization with colchicine. Comparison of the efficiency of different colchicine concentrations and duration of callus cultivation on both solid and liquid medium has been conducted. As a result, hexaploid plants regenerated after the treatment of triploid callus culture. According to the results of this study, the cultivation of calli in liquid medium containing colchicine was more efficient for obtaining polyploid calli than in solid medium. The survival of calli after culturing in liquid medium with 313 μM colchicine for 7 days was as high as 80%, however, the increase in colchicine concentration up to 939 μM drastically reduced this percentage (7.5%); in calli cultured on solid medium, these percentages were 75 and 50%, respectively. At the same time, 66.7% of calli cultured in liquid medium with 939 μM colchicine for 2 days were hexaploid, whereas the percentage of polyploids was only 44% in calli cultured on solid medium. This suggests that the antimitotic substance is absorbed by callus cells more efficiently under conditions of a liquid environment. However, the percentage of regenerated plants was very low, precisely 8.9%. Subsequently, only 2 out of the 23 plants regenerated from calli cultivated in liquid medium were hexaploid, whereas no hexaploid plants have been obtained from calli cultured on solid medium [20].
The study on colchicine polyploidization of diploid M. sacchariflorus has also been conducted. Meristems of 3 mm in length from the top of shoots were cultured on regeneration medium containing 2 g/l colchicine for 16 h in the dark without callus initiation. The survival rate of explants under these conditions was 31.2%. This study resulted in 3 tetraploid M. sacchariflorus plants, whereas the remaining plants were diploids or aneuploids. The main advantage of using shoot tips as explants and direct micropropagation on medium with antimitotic agents resides on the possibility to obtain polyploids, while avoiding somaclonal variations, since it is based on direct organogenesis [54].
3. MISCANTHUS POLYPLOIDIZATION WITH DINITROANILINES
Different works have reported both high efficiency and phytotoxicity towards both monocotyledonous and dicotyledonous plants of the most dinitroanilines used. The high toxicity of oryzalin and trifluralin has been observed on in vitro grown shoots of mint (Nepeta sp. L). Phytotoxic effect on growth and development of explants was, in this case, equally high for both compounds [34]. Similar results have been obtained in studies on the polyploidization of different Miscanthus species with oryzalin [20, 28, 29].
In order to develop a genotype-independent method for M. sinensis polyploidization, a study was conducted using oryzalin as antimitotic substance on plants grown in vivo, in vitro grown shoots and callus culture. To enhance the uptake of the antimitotic substance by tissues, the solutions contained 1.5% DMSO. Treatment of separate in vitro shoots with oryzalin at concentrations of 30, 60 and 120 µM for 18 h proved to be extremely toxic since a few explants survived only at the lowest concentrations. As a result, 2 polyploid lines have been obtained from the new shoots. With the reduction of both oryzalin concentrations (5, 10 and 15 µM) and duration of treatment (4 and 7 h), the survival of explants was significantly higher. Thus, after treatment of M. sinensis shoots with 15 µM oryzalin for 7 days, 21 out of the 26 plants obtained and analyzed were tetraploid, with an efficiency of polyploidization of 80.7%. The highest number of tetraploids obtained with 10 mM oryzalin for 4 days being 24 tetraploid plants were obtained, although the efficiency of polyploidization was only 60%. The efficiency of the treatment of M. sinensis embryogenic calli with oryzalin has also been studied. Callus culture obtained from immature inflorescences has been treated with this antimitotic for 2 or 4 days before being transferred onto plant regeneration medium. Overall, 124 plants were regenerated, however, only one of them was tetraploid [28, 29].
Another study on M. × giganteus polyploidization has revealed that oryzalin is more effective than colchicine. However, the number of regenerated plants after treatment with oryzalin (5, 10, 15 and 30 µM) for 2, 4, or 7 days was lower than the number of regenerated plants after treatment with colchicine, 8.9% and 6.0%, respectively. Explants cultured on a liquid medium supplemented with 20 μM oryzalin for 7 days were completely dead, whereas the survival rate of explants was 12.5% when the same treatment was performed on solid medium. Analysis of treated calli with flow cytometry showed direct dependence of a number of hexaploid and chimeric cells on oryzalin concentration. If treatment with 5 µM oryzalin resulted in polyploidization rate of about 20%, an increase in oryzalin concentration up to 15 µM resulted in a percentage of hexaploid and chimeric cells of 63%. Thus, after regeneration from calli treated with oryzalin, 5 out of the 10 regenerated plants were hexaploids. However, treatment with 10 µM oryzalin in liquid medium for 4 days was the most effective, since out of the 3 plants regenerated, 2 were hexaploids [20].
The results of our studies on oryzalin align with those reported above and all emphasize the high phytotoxicity of oryzalin. After cultivation of M. × giganteus calli on solid medium supplemented with 10 µM oryzalin for 14 days, the survival rate of explants did not exceed 6.7%, with only 20% of these explants being able to regenerate green plants. Similar results have been obtained on in vitro grown shoots treated for 14 days with 50 µM oryzalin or trifluralin in the medium: the survival rate of explants was about 8.3% for both compounds (unpublished data).
We also conducted experiments on in vitro grown M. sinensis shoots treated with 10 µM trifluralin in the medium for 7 or 14 days and monitored the survival rate of shoots after a month from the beginning of the treatment. This rate was 44.4% and 24.0% for explants, which experienced trifluralin for 7 and 14 days, respectively. In these experiments, oryzalin also showed higher phytotoxocity level than trifluralin. Likewise, when in vitro grown shoots of M. sinensis shoots were treated with 10 µM oryzalin for 7 and 14 days, the survival rate was 32.53% and 23.52%, respectively (unpublished data).
4. MISCANTHUS POLYPLOIDIZATION WITH NEWLY SYNTHESIZED DINITROANILINE COMPOUNDS
As reported above, the spatial structure of α-tubulin (Q70ZL7), from miscanthus, has been reconstructed and verified using in silico methods [51]. Then, the ability of known dinitroanilines (oryzalin and trifluralin) as well as newly synthesized compounds to form ligand-protein complex with miscanthus α-tubulin molecule was evaluated. The phytotoxicity of these dinitroanilines was evaluated by the Allium test [49] and the most promising newly synthesized compounds selected to be used in the polyploidization of Miscanthus [51]. These compounds are given in (Table 1).
According to our results, the application of new dinitroaniline compounds for polyploidization of M. × giganteus showed a significant decrease in phytotoxicity level compared to trifluralin and oryzalin. Thus, following the addition in the micropropagation medium of 50 µM of substances, listed in Table1, for the first 14 days, the survival rate of explants ranged from 83.3% to 91.6%, which is significantly higher than that obtained for well-known dinitroanilines (oryzalin and trifluralin) at the same concentration (8.3%) [55, 56]. Also, both new and classic dinitroanilines appeared to be capable of M. × giganteus polyploidization.
The survival rates of in vitro grown M. sinensis shoots after a month of starting the treatment with newly synthesized dinitroanilines, such as N′-(N′′-[2,6-dinitro-4-trifluoromethyl phenyl]propyl) morpholine and 1-{3-[2-(2,6-dinitro-4-trifluhyl-2-phenylamino -2,3-dihydro-thiazole-5-yl}-ethanol hydrochloride, at 10 µM concentration for 7 days were also high: being 95.6% for the former and 96.5% for the latter compound. With the increase of the treatment duration up to 14 days, the survival rate was substantially similar being 95.0% and 95.4% for N′-(N′′-[2,6-dinitro-4-trifluoromethylphenyl] propyl) morpholine and 1-{3-[2-(2,6-dinitro-4-trifluoromethyl-phenylamino)-ethyl]-4-methyl-2-phenylamino-2,3-dihydro-thiazole-5-yl}-ethanol hydrochloride, respecitively.
5. BIOLOGICAL AND BIOCHEMICAL TRAITS OF POLYPLOIDS
We have conducted a study of biological and biochemical traits of hexaploid M. × giganteus lines resulting from polyploidization with new dinitroanilines. Obtained synthetic polyploids possess 114 chromosomes (Fig. 2).
Plant height is one of the most important parameters for biomass production. According to the results of our study, the average height at the end of the first growing season was 180 cm for M. × giganteus triploid (control) plants and 200– 210 cm for M. × giganteus hexaploid lines. Also, the hexaploid lines exhibited higher levels of phytomass accumulation and dry matter content than control lines, whereas ash content was not dissimilar. Such traits are considered to be promising for the utilization of hexaploid lines as a raw material in the manufacturing of solid fuels (pellets) and in bioethanol production. Also, biochemical parameters of all obtained hexaploid lines differ significantly from the control. In particular, a single hexaploid line showed higher content of total sugars and monosaccharides than the other hexaploid lines and control plants.
According to the results of other researchers, by the end of the third year of vegetation, polyploid lines of M. × giganteus overcome triploid plants only in stem diameter, which is 12.6 and 10.4 mm, respectively. It has also been observed that the height of triploid and polyploid plants was 288 and 256.7 cm, respectively [29]. In previous studies and comparisons between polyploid and triploid M. × giganteus plants, Yu et al. (2009) observed that polyploids had a greater stem diameter and did not show a significant difference in growth rate compared to the control plants [20]. Polyploids had longer (30.0 µm) stomatal length than triploid M. × giganteus plants (24 µm) [20, 28, 29].
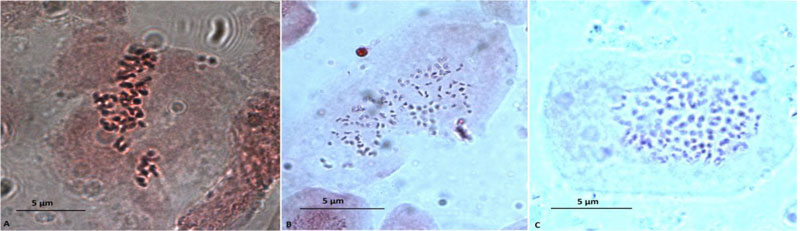
Chemical Name | Formula | |
---|---|---|
4-methylsulfonyl-2,6-dinitroaniline | C7H7N3SO6 | ![]() |
N′-(N”-[2,6-dinitro-4-trifluoromethylphenyl] propyl)morpholine | C14H17O5N4F3 | ![]() |
N,N'-bis-(2-nitro-phenyl)-hexylene-1,6-diamine | C18H22N4O4 | ![]() |
N'-[2,6-dinitro-4-(trifluoromethyl)phenyl]ethane-1,2-diamine; hydrochloride | C9H10ClF3N4O4 | ![]() |
1-{3-[2-(2,6-dinitro-4-trifluoromethyl- phenylamino)-ethyl]-4-methyl-2-phenylamino-2,3-dihydro-thiazole-5-yl}-ethanol hydrochloride | C21H19ClF3N5O5S | ![]() |
{2-[4-(2,4-dichlorophenyl)-2-phenylamino-thiazole-3-yl]-ethyl}-(2,6-dinitro-4- trifluoromethyl-phenyl)-amine hydrochloride | C24H17Cl3F3N5O4S | ![]() |
CONCLUSION
M. × giganteus is a sterile allotriploid that has great potential for biofuel production [57-59]. One of the critical factors determining the efficiency of antimitotic substances in inducing polyploidy is their toxicity to the plant. It has been found that the application of colchicine at low concentration (i.e. 313 µM) resulted in the highest number of polyploids obtained and ensured a high percentage of explant survival after treatment of both calli and shoots in vitro [52, 29]. Petersen et al. (2002) confirmed that the highest number of tetraploid plants can be obtained with a low concentration (156 µM) of colchicine on in vitro grown shoots [28]. Studies on M. × giganteus polyploidization have shown that oryzalin is more effective than colchicine. However, almost the complete death of explants was observed when M. × giganteus explants were treated with oryzalin and only 2 tetraploid lines were obtained. According to this study, the use of oryzalin in a liquid medium was more effective than colchicine treatment, however, high phytotoxicity of the former had a negative effect on explants survival rate and, as a result, on the number of induced polyploids. In particular, oryzalin treatment inhibited the regeneration of plants from calli [20]. A similar situation has been observed in the study of corn polyploidization, where the treatment with oryzalin decreased the percentage of calli able to regenerate plants compared to treatment with trifluralin and APM [47]. The results of our studies are in agreement with those of other researchers, highlighting the problems with high phytotoxicity of classic dinitroanilines on M. × giganteus and M. sinensis. It has been found that in vitro treatment of rooted plants is ineffective since only a small proportion of tetraploids and a large number of chimeric lines were obtained [29]. Application of new in silico selected compounds for polyploidization of M. × giganteus showed a significant decrease in phytotoxicity level compared with trifluralin and oryzalin and, as a consequence, in a higher survival rate of the treated explants. Newly synthesized dinitroanilines, such as 4-methylsulfonyl-2,6-dinitroaniline, N′-(N”-[2,6-dinitro-4-trifluorome thylphenyl]propyl)morpholine,N,N'-bis-(2-nitro-phenyl)-hexy lene-1,6-diamine, N’1’-(2,6-dinitro-4-trifluoromethyl -phenyl)-ethane-1,2-diamine hydrochloride, 1-{3-[2-(2,6-dinitro-4-trifluo romethyl- phenylamino)-ethyl]-4-methyl2-phenylamino-2,3-dihydro-thiazole-5-yl}-ethanol hydrochloride and 24-(2,4-dichlorophenyl)-2-phenylamino-thiazole-3-yl]-ethyl}-(2,6-dinitro-4-trifluoromethyl-phenyl)-amine hydrochloride, are able to induce polyploidization in M. × giganteus as well as classic dinitroanilines. Polyploids differ significantly from their original forms in biological and biochemical traits. However, problems with fertility restoration of M. × giganteus have not been solved yet and require further efforts.
CONSENT FOR PUBLICATION
Not applicable.
FUNDING
This research was carried out within the framework of R&D projects “Creation of new high-yielding lines of Miscanthus as a raw material for bioethanol via obtaining of polyploids” (0113U004719) of R&D Programme of Natl. Academy of Sci. of Ukraine “Biological Resources and New Technologies of Bioconversion” (2013-17) and “Development of high-yielding polyploid lines of Miscanthus as a raw materials for bioethanol production and characterization of their productivity” (0118U005354) of R&D Program of the Natl. Acad. of Sci. of Ukraine “Biofuels and Bioenergy” (2018-19).
CONFLICT OF INTEREST
The authors declare no conflict of interest, financial or otherwise.
ACKNOWLEDGEMENTS
We acknowledge Dr. V. Kyrylenko (Institute of Food Biotechnology and Genomics, National Academy of Sciences of Ukraine, Kyiv) suggestions aimed to improve the manuscript language.