All published articles of this journal are available on ScienceDirect.
Antibacterial and Antioxidant Activities of Fomitopsis betulinaExtracts
Abstract
Background
The Birch polypore fungus (Fomitopsis betulina), a well-known brown-rot macromycete parasitizing Betula spp., is recognized as a medicinal mushroom due to its antimicrobial, antiviral, antioxidant, anti-inflammatory, anticancer, and other bioactivities. Recently, mushrooms have been explored as potential therapeutic agents in veterinary medicine and livestock farming, suggesting that F. betulina could contribute significantly to these fields.
Objective
This study aimed to evaluate the antibacterial and antioxidant activities, as well as the phenolic compounds content, in ethyl acetate extracts of 22 F. betulina strains.
Methods
The agar well diffusion method was used to assess antibacterial activity. Antioxidant activity and total phenolic content (TPC) were measured spectrophotometrically using the 2,2-diphenyl-1-picrylhydrazyl (DPPH) assay and the Folin-Ciocalteu method, respectively.
Results
All 22 F. betulina strains exhibited antibacterial and antioxidant activities, as well as phenolic compound content, with variations attributed to strain-specific characteristics. The fungal extracts demonstrated susceptibility against Bacillus subtilis, Escherichia coli, Klebsiella pneumoniae, Pseudomonas aeruginosa, and Staphylococcus aureus. Zones of bacterial growth inhibition ranged from 8.0±0.0 mm to 22.5±0.5 mm. The free radical scavenging activity varied from 7.74±2.40% to 96.66±0.40%. TPC ranged from 0.01±0.00 to 8.57±0.18 mg GAE/g of dry sample.
Conclusion
Ethyl acetate extracts derived primarily from the mycelia of F. betulina strains were identified as particularly beneficial, with strains F. betulina 2777 and 2778 emerging as promising biotechnological producers. F. betulina mycelium has a wide potential for human use, including as feed additives for livestock and the development of veterinary therapies.
1. INTRODUCTION
Fomitopsis betulina (Bull.) B.K. Cui, M.L. Han, and Y.C. Dai is a widely distributed brown rot macrofungus, particularly prevalent in the northern temperate zones of Europe, North America, and Asia, primarily found on dead and living trees of Betula spp. The fruiting bodies of this species have been used in folk medicine for centuries. Modern studies have confirmed its anticancer, anti-inflammatory, antiviral, antimicrobial, antioxidant, antimalarial, and other biological activities. [1-3].
In addition to research into the medical uses of mushrooms, there is also growing interest in cosmetology and veterinary applications. These areas have formed the foundation of medicinal mushroom studies at the Jagiellonian University Medical College over the past thirty years [4]. Derkach and Klymenko [5] conclude that pharmacokinetics and pharmacodynamics studies on the biologically active metabolites of basidiomycetes provide new opportunities for the scientifically grounded use of certain mushroom species in veterinary medicine.
The review of the cytotoxic activity of F. betulina against normal and cancer cells suggests that extracts, especially ethanolic and methanolic, exhibit selective cytotoxicity against cancer cells while the impact on healthy cells is minimal. The strongest effects were observed in prostate cancer and melanoma cell lines. The cytotoxic effects were largely attributed to triterpenes and glucans [6]. Sułkowska-Ziaja et al. [7] showed cytotoxic and anti-inflammatory activity of F. betulina extracts from fruiting bodies and mycelial cultures. The anti-inflammatory activity of fruiting bodies and mycelium extracts had been evaluated based on their inhibition of pro-inflammatory enzymes. Sevindik et al. [8] collected information about F. betulina antiviral activity against the Herpes virus, Influenza, Poliovirus, and Vesicular stomatitis virus.
Developing new antibacterial drugs is a pressing need due to the continuous emergence of pathogens that are multi-resistant to existing antibiotics, as well as new types of pathogens. Researchers are especially interested in drugs derived from natural sources that exhibit antibacterial activity and have minimal side effects. Consequently, the antibacterial potential of higher fungi is attracting growing interest [9, 10].
Krupodorova et al. [11] examined the antibacterial activities of thirty mushroom species (F. betulina among them), cultivated in two liquid media. The study found that the antibacterial potential of the culture liquids from these species was higher than the activity of their mycelia. F. betulina demonstrated high antibacterial efficiency, which was compared with that of certain commercial antibiotics and natural essential oils of Salvia and Eucalyptus. The culture liquid of F. betulina shows potential for use in the development of antibacterial products. Various preparations of F. betulina culture liquid, including native, native concentrated, lyophilized, and dried forms, were assessed against a range of standard bacterial strains and clinical isolates [12], highlighting the potential of F. betulina in antimicrobial applications.
An equally important area of research is the antioxidant potential of mushrooms. Reactive oxygen species (ROS) and reactive nitrogen species (RNS) are free radicals capable of destroying healthy cells in the body. An imbalance in the formation of free radicals as a result of overproduction is known as oxidative stress, which is a major factor in ageing and the development of a number of degenerative diseases in humans [13].
Vunduk et al. [14] tested F. betulina hot water (HW), partially purified (PP), and alkali (HA) extracts for antioxidant activity. HA was a good 1,1-diphenyl-2-picrylhydrazyl radical scavenger and exhibited the strongest ferric-reducing power. Among other fungal species, F. betulina has also been mentioned as a source of antioxidants in a research [13]. The researcher [3] collected data on 109 secondary metabolites and various small molecules found in F. betulina fruiting bodies (96 compounds) and mycelial fermentation broth (13 compounds). The authors described the therapeutic activity of these compounds: anticancer, anti-inflammatory, antimicrobial, and antioxidant properties. The importance of the process of secondary metabolite biosynthesis in higher fungi has been evident.
It is important to consider that research should focus not only on the fruiting bodies of mushrooms, as is common, but also on other vegetative forms and products of their cultivation, such as mycelia and culture liquids. Studies that allow for both the evaluation and comparison of these different forms are particularly valuable.
Consequently, information on the antimicrobial and antioxidant properties of F. betulina mycelium is scarce. It is important to note that different strains of the same mushroom vary in their properties [15-18].
The study aimed to evaluate 22 different F. betulina strains for their antibacterial and antioxidant activities in mycelial and culture liquid extracts, as well as to measure their total phenolic content.
2. MATERIALS AND METHODS
2.1. Fungal and Bacterial Cultures
Twenty-two F. betulina strains (under the numbers F. betulina 311, 327, 978, 988, 989, 2269, 2290, 2363, 2364, 2366, 2399, 2770, 2771, 2772, 2773, 2774, 2775, 2776, 2777, 2778, 2785, and 2786) were provided by the IBK Mushroom Culture Collection of the M.G. Kholodny Institute of Botany of the National Academy of Sciences of Ukraine [19]. All fungal cultures were stored at 4°C on the malt extract agar (MEA, Thermo Fisher Scientific, USA) slants.
Bacterial cultures (Bacillus subtilis, Escherichia coli, Klebsiella pneumoniae, Pseudomonas aeruginosa, and Staphylococcus aureus) were generously supplied by the Collection of microorganisms of the M.G. Kholodny Institute of Botany of the National Academy of Sciences of Ukraine and were used for further investigation of antibacterial activity. These strains were maintained on the Mueller-Hinton Agar (MHA, Sanimed-M, Ukraine) slants at 4°C.
2.2. Cultivation Conditions and Preparing of Extracts
Glucose peptone yeast agar (GPYA) containing g/L: 25.0 g glucose (Lycadex® PF, France), 2.0 g yeast extract (Conda, Spain), 3.0 g peptone (Conda, Spain), 1.0 g K2HPO4 (Hebei Ruisite Technology Co., Ltd, China), 1.0 g KH2PO4 (Haifa Group, Israel), 0.25 g MgSO4·7H2O (Poluks Group, Poland), and 20.0 g agar (Conda, Spain); and the same medium without agar – glucose peptone yeast broth (GPYB) were used for F. betulina cultivation. All prepared media were autoclaved at 121°C for 15 min.
The inoculum of each F. betulina strain was carried from slants to GPYA Petri dishes and then incubated at 25±1°C in the dark with ventilation for 10 days. Afterward, 3 mycelial disks 8 mm in diameter from 10-day cultures of F. betulina strains were inoculated in each flask with GPYB and incubated at 25±1°C for 14 days in the method of surface liquid static cultivation.
The mycelia and culture liquid of each strain were separated by filtration using Whatman No 4 filter paper. Mycelia were washed twice with distilled water and dried at 60°C to constant weight.
The dry mycelia of each F. betulina strain were extracted by adding ethyl acetate (Carlo Erba, Italy) at a 1:10 (w/v) ratio. The mixtures were shaken for two days at 100 rpm and 30°C. Extracts were then separated from the mycelia by centrifugation at 4000 rpm for 10 minutes, followed by filtration using Whatman No. 4 filter paper. The culture liquid of each strain was extracted by adding ethyl acetate at a 2:1 (v/v) ratio. The mixtures were kept at 8°C for two days and then separated using a separatory funnel. Ethyl acetate fractions were concentrated to dry mass using a rotary evaporator (RVO-64, Czechoslovakia) at 40°C. The dry weight of each extract was determined, and each dried extract was dissolved in 5 mL of ethyl acetate.
2.3. Evaluation of Antibacterial Activity (ABA)
The tested microorganisms (B. subtilis, E. coli, K. pneumoniae, P. aeruginosa, and S. aureus) were activated in Mueller Hilton agar (MHA) at 37°C for 24 hours. Each microorganism was suspended in sterile saline and diluted to 106 colony-forming units (CFU) per ml. Petri dishes with MHA were then inoculated with 0.5 ml of the bacterial suspensions. The antibacterial activity was determined by the agar well diffusion method. Holes with a diameter of 7 mm were aseptically cut out in the agar layer of previously inoculated Petri dishes using a sterile cork borer. A total of 120 µL of each F. betulina strain's mycelial and culture liquid extract was aseptically pipetted into the wells. Then, agar plates were incubated at 37°C for 24 h.
The antibacterial activity was determined by measuring the inhibition zone diameter (mm), which appeared as clear zones around each well. The activity was detected when the inhibition zone exceeded 7 mm. Ethyl acetate, used for extraction, served as the negative control.
2.4. Evaluation of Antioxidant Activity (AOA)
AOA was determined using the 2,2-diphenyl-1-picrylhydrazyl (DPPH) method (Thermo Fisher Scientific, USA) [20]. The analysis was performed spectrophotometrically (UV-1800 PC, Shanghai, China) following the protocol [17]. Ascorbic acid (Thermo Fisher Scientific, USA) was used as the positive control.
2.5. Evaluation of Total Phenolic Content (TPC)
Phenolic compounds in prepared extracts were determined spectrophotometrically by the Folin-Ciocalteu method [21]. 0.5 ml of each mycelial and culture liquid extract were transferred into 25 ml volumetric flasks. Then, 2.0 ml of 10% water Folin-Ciocalteu (Carl Roth, Germany) solution was added. After a few minutes, 10.0 ml of 7.5% water solution of Na2CO3 (Thermo Fisher Scientific, USA) were loaded in flasks, and all reaction mixtures were filled up to 25 ml with water. After 40 min of exposition, the prepared solutions were measured at a wavelength of 750 nm. The mixture containing 2.0 ml of Folin-Ciocalteu solution and 10.0 ml of 7.5% Na2CO3 solution, diluted to a final volume of 25 ml, was used as a blank. The content of phenolic compounds was calculated according to the calibration curve with gallic acid (TCI America™, USA) as a standard (y = 0,0758x + 0,0583; R2 = 0,9685).
Gallic acid concentrations ranging from 2 to 40 μg/ml were used. The determined TPC values are expressed as milligrams of gallic acid equivalent (GAE) per gram of dry sample (dry mycelia or dry culture liquid extract).
2.6. Statistical Analysis
All assays were performed in five replicates to calculate the mean value. Results were presented as means ± standard deviation. Pearson correlation coefficients (r) were calculated for ABA, AOA, and TPC, as well as for biomass and exopolysaccharide (EP) production determined in a previous study [18], using the online statistical software available at https://www.statskingdom.com/. Statistical analysis was conducted using Fisher’s LSD test, with differences considered significant at P < 0.05.
3. RESULTS
3.1. ABA of F. betulina Strains
All of the investigated F. betulina strains (100%) demonstrated the presence of ABA in mycelial and/or culture liquid extracts (Table 1). The zone of bacterial growth inhibition varied from 8.0±0.0 mm to 22.5±0.5 mm in diameter.
The screening identified F. betulina strains with the highest levels of bacterial growth inhibition. Notably, strain 978 exhibited maximum inhibition against B. subtilis, E. coli, and K. pneumoniae, while strain 2269 demonstrated specific activity against P. aeruginosa. All mycelial extracts (100%) exhibited varying levels of ABA, while 90.9% of culture liquid extracts were active, except for F. betulina 2770 and 2772 (Figs. 1 and 2). Regarding the spectrum of activity and frequency of occurrence, mycelial extracts demonstrated higher ABA rates than culture liquid extracts.The test bacteria B. subtilis and K. pneumoniae were more susceptible to mycelial extracts, with inhibition observed in 95.5% and 90.9% of samples, respectively (Fig. 1). E. coli and S. aureus also showed considerable sensitivity to both culture liquid and mycelial extracts.
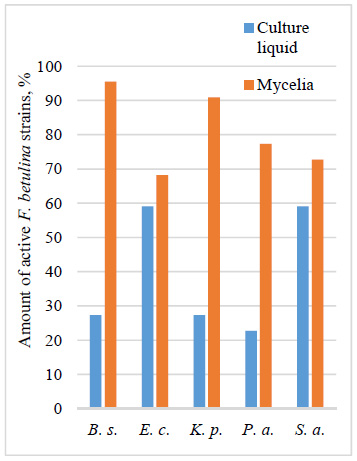
Activity of F. betulina strains against each of the test bacteria. Note: B. s. – B. subtilis, E. c. – E. coli, K. p. – K. pneumoniae, P. a. – P. aeruginosa, S. a. – S. aureus.
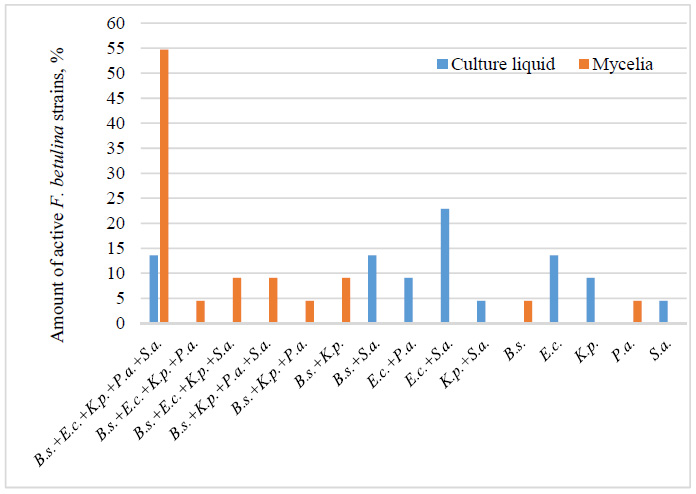
Antibacterial spectra of F. betulina strains. Note: B.s.+E.c.+K.p.+P.a.+S.a. – inhibition of growth of all bacteria: B. subtilis, E. coli, K. pneumoniae, P. aeruginosa, S. aureus; B.s.+E.c.+K.p.+P.a., B.s.+E.c.+K.p.+S.a., B.s.+K.p.+P.a.+S.a. – inhibition of growth of four respective bacteria; B.s.+K.p.+P.a. – inhibition of growth of three respective bacteria; B.s.+K.p., B.s.+S.a., E.c.+P.a., E.c.+S.a., K.p.+S.a. – inhibition of growth of two respective bacteria; B.s., E.c., K.p., P.a., S.a. – inhibition of growth of one respective bacteria.
The broadest spectrum of activity against all five tested bacteria, in both mycelial and culture liquid extracts, was observed in F. betulina strains 978 and 2778. Additionally, mycelial extracts of F. betulina exhibited broader activity, inhibiting five, four, or three bacteria in 81.9% of fungal strains, while 18.1% were active against two or one pathogen. In contrast, culture liquid extracts showed narrower activity, with 86.4% of strains inhibiting two or one bacterium and only 13.6% affecting all tested bacteria.
3.2. AOA of F. betulina Strains
The AOA of F. betulina strains was assessed as the percentage of free radical inhibition. All investigated samples (100%) demonstrated DPPH radical scavenging activity (Fig. 3). The AOA of culture liquid extracts ranged from 18.32±0.50% to 96.66±0.40%, while mycelial extracts exhibited activity levels between 7.74±2.40% and 90.95±0.60%. The highest AOA values were observed in F. betulina strain 2773 for culture liquid and strain 988 for mycelium. Moreover, the culture liquid extract of F. betulina 2773 showed activity comparable to the positive control, ascorbic acid (95.97±0.30%). Among F. betulina strains, 68.2% showed higher activity in culture liquid than in mycelium, while 31.8% exhibited greater activity in mycelium than in culture liquid. However, regarding free radical scavenging percentages, an AOA rate of ≥70% was observed more frequently in mycelial samples (36.6% of all fungal strains) compared to culture liquid samples (22.7%).
In addition, F. betulina strains 2777 and 2778 exhibited relatively similar activity, with strain 2777 showing 90.86±0.60% in culture liquid and 81.37±0.50% in mycelium, while strain 2778 demonstrated 91.02±0.45% and 79.61±0.50%, respectively. These values are considered relatively high in both mycelial and culture liquid extracts.
Strain | Zone of inhibition by culture liquids extracts, mm in diameter | Zone of inhibition by mycelial extracts, mm in diameter | ||||||||
---|---|---|---|---|---|---|---|---|---|---|
B. subtilis | E. coli | K. pneumoniae | P. aeruginosa | S. aureus | B. subtilis | E. coli | K. pneumoniae | P. aeruginosa | S. aureus | |
311 | 10.0±0.0с | 16.5±0.5ab | 14.5±0.5b | 14.5±0.5c | 14.5±0.5d | 17.5±0.2bd | – | 13.0±0.0f | 11.5±0.5e | 8.0±0.0hg |
327 | – | 14.0±0.0d | – | 13.0±0.0c | – | 10.0±0.0j | 8.5±0.5g | 18.0±0.0b | 9.5±0.5f | 8.5±0.5g |
978 | 12.0±1.0a | 15.5±0.5bc | 16.0±1.0a | 18.0±1.0b | 17.5±0.5c | 18.0±0.4b | 15.0±1.0c | 15.0±0.0d | 15.0±1.0cd | 18.5±0.5b |
988 | – | 10.0±0.0h | – | – | 18.0±0.0c | 17.0±0.2de | 15.5±0.5c | 21.5±0.5a | 15.0±1.0cd | 16.5±0.2c |
989 | – | 10.0±0.0h | – | – | 10.0±0.0e | 9.0±0.0k | – | 9.5±0.5i | 9.0±0.0g | – |
2269 | – | 17.0±0.0a | – | 20.0±0.0a | – | 16.0±1.0ef | 16.0±0.3c | 17.0±0.4c | 19.0±0.3a | 12.5±0.5f |
2290 | – | 13.0±0.0e | – | – | 22.0±0.0b | 14.0±0.0h | 14.0±0.0d | 15.0±1.0cd | 14.5±0.5d | 18.5±0.5b |
2363 | – | 11.0±0.0g | – | – | 10.0±0.0e | 14.0±0.0h | 19.0±1.0ab | 15.0±1.0cd | 17.0±0.2b | 20.5±0.5a |
2364 | – | – | 9.0±0.0d | – | – | 13.5±0.5hi | – | 13.0±0.0е | – | – |
2366 | – | 12.0±0.0f | – | – | – | 17.5±0.5b | 14.0±1.0cd | 14.0±1.0de | 16.5±0.5bc | 14.0±1.0e |
2399 | 9.0±0.0d | – | – | – | 10.0±0.0e | 21.0±0.4a | 19.0±0.3b | 16.0±1.0c | 15.0±0.0d | 16.0±0.2d |
2770 | – | – | – | – | – | 17.5±0.5b | 8.0±1.0fg | 15.0±0.0d | 15.0±1.0cd | – |
2771 | – | 10.0±0.0h | – | – | – | 8.5±0.5k | 14.0±0.0d | 21.0±0.3a | – | 14.5±0.5e |
2772 | – | – | – | – | – | 13.0±0.0i | – | 13.0±1.0e | – | – |
2773 | – | – | 9.5±0.5c | – | 10.0±0.0e | 14.0±1.0gh | 12.5±0.5e | 12.5±0.5ef | 14.5±0.5d | 15.0±0.5e |
2774 | 11.0±0.0ab | – | – | – | 10.0±0.0e | 16.0±0.4f | 10.0±0.0f | 11.5±0.5fg | – | 9.0±0.0g |
2775 | – | – | – | – | 10.0±0.0e | 15.5±0.5fg | – | – | – | – |
2776 | – | – | 9.0±0.0d | – | – | 20.0±1.0a | 9.5±0.5f | 15.0±0.3d | 16.0±1.0bc | 11.0±1.0f |
2777 | – | 10.0±0.0h | – | – | 10.0±0.0e | 14.0±0.0h | 13.0±0.0e | 14.0±1.0de | 16.0±0.2c | 14.5±0.5e |
2778 | 9.0±0.0d | 9.0±0.0i | 9.0±0.0d | 10.0±0.0d | 9.0±0.0f | 16.0±0.3f | 20.5±0.5a | 18.0±0.3b | 15.0±0.0d | 16.5±0.5c |
2785 | 12.0±0.0а | – | – | – | 22.5±0.5a | 8.0±1.0k | – | 17.0±1.0bc | 12.5±0.5e | 9.0±0.0g |
2786 | – | 12.0±0.0f | – | – | – | – | – | – | 10.0±0.0f | – |
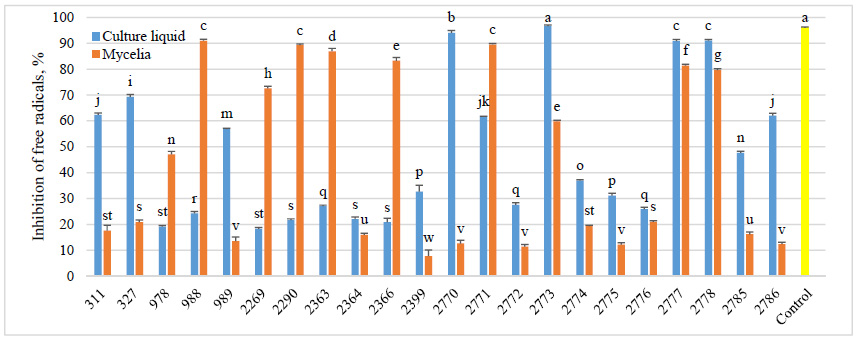
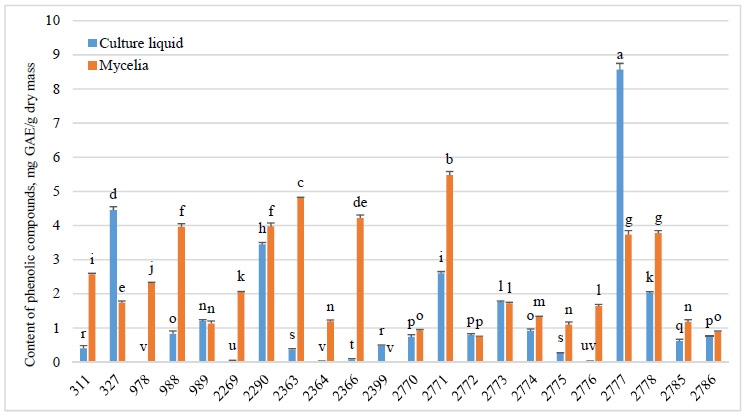
Correlation | Culture liquid | Mycelia | |
---|---|---|---|
ABA/AOA | B. subtilis/AOA | -0.01225 | 0.11440 |
E. coli/AOA | -0.06057 | 0.72470 | |
K. pneumoniae/AOA | 0.06948 | 0.49270 | |
P. aeruginosa/AOA | -0.03707 | 0.41580 | |
S. aureus/AOA | -0.09897 | 0.76190 | |
ABA/TPC | B. subtilis/TPC | -0.21320 | 0.03796 |
E. coli/TPC | 0.21280 | 0.56180 | |
K. pneumoniae/TPC | -0.23030 | 0.46800 | |
P. aeruginosa/TPC | -0.06924 | 0.24010 | |
S. aureus/TPC | 0.07679 | 0.66500 | |
ABA/EP (for culture liquid) | B. subtilis/EP | 0.24700 | – |
E. coli/EP | -0.22640 | – | |
K. pneumoniae/EP | -0.09976 | – | |
P. aeruginosa/EP | 0.02700 | – | |
S. aureus/EP | 0.04268 | – | |
ABA/biomass (for mycelia) | B. subtilis/biomass | – | 0.08667 |
E. coli/biomass | – | -0.47760 | |
K. pneumoniae/biomass | – | -0.03541 | |
P. aeruginosa/biomass | – | -0.18350 | |
S. aureus/biomass | – | -0.37730 | |
AOA/TPC | 0.52030 | 0.90510 | |
AOA/EP (for culture liquid) | 0.10150 | – | |
AOA/biomass (for mycelia) | – | -0.43240 | |
TPC/EP (for culture liquid) | 0.23430 | – | |
TPC/biomass (for mycelia) | – | 0.90510 |
3.3. TPC of F. betulina Strains
Phenolic compounds were detected in all (100%) F. betulina strains (Fig. 4). TPC in culture liquid extracts ranged from 0.01±0.00 to 8.57±0.18 mg GAE/g of dry extract, while in mycelial extracts, it varied from 0.01±0.00 to 5.48±0.10 mg GAE/g of dry mycelium. The highest TPC values were observed in the mycelial extract of F. betulina strain 2771 and the culture liquid extract of strain 2777.
In 72.7% of F. betulina strains, TPC was higher in mycelia than in culture liquid, while only 13.6% of strains had higher TPC values in culture liquid. Strains F. betulina 989, 2772, and 2773 showed no statistically significant differences in phenol content between culture liquid and mycelium. Additionally, TPC values in culture liquid were more variable than in mycelial content.
3.4. Correlation between ABA, AOA, TPC, EP, and Biomass
The Pearson coefficient (r) was calculated to reveal the potential correlation between ABA, AOA, and TPC in F. betulina strains (Table 2). In addition, the relationship between the obtained results and the biomass and extracellular polysaccharide (EP) production of the same F. betulina strains on GPDB medium was investigated, with data presented in our previous study [18].
No significant dependence was found for values gained for culture liquid, except for AOA and TPC levels, which showed a strong positive correlation. In contrast, mycelial parameters showed more interrelations. A strong positive correlation was observed between AOA and TPC (similar to culture liquid), as well as between TPC and biomass synthesis. Positive correlations of varying strength were detected between TPC and ABA, and between AOA and ABA. Specifically, ABA against E. coli, K. pneumoniae, and S. aureus was significantly associated with AOA and TPC levels, as was ABA against P. aeruginosa with AOA. A strong positive correlation was also found between TPC and biomass amounts.
Additionally, a negative correlation was noted between biomass synthesis levels and biological activities, with a medium dependence for AOA and ABA against E. coli and S. aureus and a weaker dependence for ABA against K. pneumoniae and P. aeruginosa.
4. DISCUSSION
Mushrooms are recognized as natural analogues of chemical therapeutic agents. The antimicrobial, antitumor, anti-inflammatory, antioxidant, and other therapeutic activities of higher fungi are well-documented and extensively studied. The identification, isolation, and purification of biologically active compounds from fungi are key objectives of numerous studies [1–3, 7, 9, 13]. Mushrooms are increasingly recognized for their antioxidant and antimicrobial activities due to their rich bioactive compounds, including phenolics, polysaccharides, terpenoids, and flavonoids, which help combat oxidative stress and inhibit microbial growth [1–3, 9, 10, 13]. This presents potential applications in health, medicine, veterinary science, and the food industry (e.g., preservation), aligning with the increasing demand for natural and sustainable solutions. Animal husbandry and veterinary medicine face ongoing challenges, including antibiotic-resistant bacteria, farm animal mortality, productivity enhancement, and animal health support. The use of edible mushrooms for animal feed and supplementation is currently being studied and applied. Various fungal compounds have demonstrated therapeutic properties: polysaccharides enhance immune response, support gut microbiome health, lower cholesterol levels, and resist avian digestive enzymes, while phenols improve the antioxidant status of chickens [4, 5, 22]. In addition, certain antibiotics, such as pleuromutilin, have been isolated from the macrofungi Omphalina mutila (formerly Pleurotus mutilus) and Clitopilus passeckerianus (formerly P. passeckerianus). Pleuromutilin derivatives, tiamulin and valnemulin, are used in veterinary medicine for pigs and poultry [23]. This underscores the importance of exploring basidiomycetes as potential sources of natural antibiotics and antioxidants. The antibacterial, antioxidant actions and phenolic compounds of F. betulina have been previously determined in numerous studies [1-5, 7, 8, 10-14, 24]. Most studies on Birch polypore fungus focus on wild fruiting bodies for their ABA, AOA, and TPC content [7, 24-29, 30]. Limited data are available on artificial cultivation, with liquid-submerged dynamic cultivation being the preferred method [7, 31, 32]. In contrast, our study utilized the liquid surface static culture method. Our results confirmed the presence of ABA, AOA and phenolic compounds in products (culture liquids and mycelia) of the artificial cultivation of Birch polypore fungus.
The ABA of F. betulina 327 strain from IBK [19], used in our research, was previously investigated with other bacterial strains [11, 12, 33, 34]. These studies mainly focused on the crude mycelia and culture liquids prepared in different methods but without extraction.
For instance, Krupodorova et al. [11] examined ABA in crude concentrated culture liquid and freshly ground mycelia cultivated on GPYB and amaranth flour-based media. In particular, crude concentrated culture liquids of F. betulina IBK 327, based on GPYB and amaranth flour, showed significant ABA against B. subtilis and S. aureus (inhibition zones ≥19 mm), and the amaranth flour-based culture liquid showed the same activity against E. coli.
In contrast, our ethyl acetate extract from the culture liquid of this fungal strain, grown on GPYB, exhibited no activity against B. subtilis and S. aureus. A comparison of ABA against E. coli revealed that the extract’s activity (inhibition zone: 14.0±0.0 mm) was slightly lower than that of the amaranth flour-based culture liquid but higher than that of the crude GPYB culture liquid. These results can be explained by selective extraction with ethyl acetate and differences between the bacterial strains used. In addition, the crude culture liquid has a wider range of extracellular metabolites with a greater potential for biological activity, some of which are not extracted with ethyl acetate. It has been reported that the total indicators of biological activity of substances contained in crude extracts and mushroom products can be higher than those of isolated compounds due to synergistic interactions between different substances synthesized by mushrooms [1].
When comparing the ABA of mycelia, our ethyl acetate extract of F. betulina 327 exhibited a broader antibacterial spectrum, demonstrating activity against all five tested cultures. In contrast, the crude ground mycelia in the referenced study [11] were effective against only B. subtilis and E. coli after cultivation in a nutrient medium based on amaranth flour. However, the ABA levels against B. subtilis and E. coli were comparable to those in our study. The zone of inhibition against both bacteria was estimated to be 10.0±0 mm against both B. subtilis and E. coli in the case of crude mycelia, 10.0±0.0 mm against B. subtilis and 8.5±0.5 mm against E. coli with ethyl acetate extracts. Despite similar ABA levels, crude mycelia likely require additional concentration steps, while ethyl acetate extraction effectively concentrates bioactive compounds.
Compared to extracts, fresh fungal materials are less suitable for storage due to spoilage risks and low metabolite concentrations, necessitating extraction, drying, or other processing methods. Krupodorova et al. [12] demonstrated the use of dry culture liquid from F. betulina IBK 327, cultivated on a modified GPYB medium. Minimum bactericidal concentrations (MBC) were tested against standard bacterial strains (S. aureus ATCC 25923, E. coli ATCC 25922, and P. aeruginosa ATCC 27853). While MBC decreased with increasing concentration (native culture liquid to dried), E. coli ATCC 25922 showed greater resistance to dried compared to lyophilized or native liquid. This variability could be attributed to heat sensitivity during drying or hygroscopicity in freeze-dried materials.
Notably, both concentrated native and dried culture liquid exhibited bactericidal effects against clinical isolates resistant to certain antibiotics. In comparison, our ethyl acetate extracts demonstrated activity against E. coli and K. pneumoniae but lacked ABA against P. aeruginosa and S. aureus. These findings highlight the need to optimize concentration and extraction methods to enhance ABA for individual fungal cultures.
Culture liquids from artificial cultivation have been analyzed. Suay et al. [31] studied methanolic culture liquid extracts from four F. betulina strains cultivated under submerged dynamic conditions. Three strains (F-048,838, F-051,849, and F-054,152) showed high activity against P. aeruginosa and Serratia marcescens (zones ≥15 mm). All strains inhibited B. subtilis growth, with three strains exhibiting inhibition zones ≥15 mm and one strain showing a zone <15 mm. Strain F-048,838 demonstrated moderate (<15 mm) activity against S. aureus. Weak ABA was detected against Mycobacterium smegmatis (strains F-048,838 and F-054,152) and S. aureus (strain F-054,152). Our ethyl acetate culture liquid extracts showed some similarity in ABA to the referenced study. F. betulina strains 311, 978, and 2269 exhibited analogous activity against P. aeruginosa, with inhibition zones of 14.5±0.5 mm, 18.0±1.0 mm, and 20.0±0.0 mm, respectively. F. betulina 978, 2774, and 2785 also demonstrated intermediate ABA against B. subtilis, similar to strain F-051,849 from the study. Some of our cultures exhibited stronger activity against S. aureus compared to strain F-048,838: F. betulina 311 (14.5±0.5 mm), 978 (17.5±0.5 mm), 988 (18.0±0.0 mm), 2290 (22.0±0.0 mm), and 2785 (22.5±0.5 mm). Weak activity against S. aureus, comparable to strain F-054,152, was observed in F. betulina 989, 2363, 2773, 2774, 2775, 2777, and 2778. These findings suggest that both submerged dynamic and surface static cultivation can support the synthesis of extracellular antibacterial compounds. Ethyl acetate extracts have also been highlighted in previous research. Schlegel et al. [32] identified a novel antibiotic, piptamine, from the F. betulina Lu 9-1 culture liquid obtained through submerged dynamic cultivation. Piptamine demonstrated high effectiveness, with a minimum inhibitory concentration (MIC) of 0.78‒12.5 μg/mL against B. subtilis, E. coli, Enterococcus faecalis, and S. aureus. In our study, strains such as F. betulina 311, 978, and 2778 exhibited broad-spectrum activity, suggesting that ethyl acetate extraction and strain selection could further enhance antibacterial compound discovery.
The biosynthesis of target compounds can also be enhanced through mutagenesis. UVC irradiation at 254 nm increased ABA in F. betulina IBK 327 culture liquid, particularly against E. coli, at an optimal dose of 0.85 kJ/cm2. However, ABA against B. subtilis and S. aureus decreased with higher doses [33]. This approach highlights the potential of physical mutagenesis to modify bioactivity based on targeted conditions.
During our ABA investigation of F. betulina strains, the tested cultures demonstrated greater susceptibility to mycelial extracts. Consequently, cultivated mycelial products were considered more effective as antibacterial agents. Among the strains, F. betulina 978 and 2778 exhibited the broadest spectrum of activity. Additionally, F. betulina 2269 strain showed specific activity against P. aeruginosa , with both its mycelia and culture liquid deemed suitable for use.
An example of ethyl acetate extraction from fruiting bodies has also been effective for isolating lanostane triterpenes, including 3β-acetoxy-16-hydroxy-24-oxo-5α-lanosta-8-ene-21-oic acid, polyporenic acids, betulinic acid, ergosterol peroxide, and fomefficinic acid [ 24 ]. These compounds displayed varying levels of ABA, primarily low or trace activity. Our ethyl acetate mycelial extracts were more effective, with inhibition zones ranging from 8.0±0.0 to 22.5± 0.5 mm. Possibly, the complex of compounds in extracts could be more efficient than one separate compound.
The AOA of our tested extracts varied widely, ranging from 7.74±2.40% to 96.66±0.40%. Notably, mycelial samples were more frequently observed as highly efficient. An AOA rate of ≥70% was detected in mycelial extracts of F. betulina strains 988, 2269, 2290, 2363, 2366, 2771, 2777, and 2778, as well as in culture liquid extracts of F. betulina strains 327, 2770, 2773, 2777, and 2778. These findings indicate that F. betulina strains 2777 and 2778 were the most likely to produce antioxidant compounds in mycelium and synthesize them in culture liquid. Other studies often determine IC50 values to assess the antioxidant activity (AOA) of F. betulina extracts, primarily using fruiting bodies [27–29]. In addition to the DPPH assay, other methods such as 2,2'-azino-bis(3-ethylbenzothiazoline-6-sulfonic acid) (ABTS), reducing power, ferric reducing antioxidant power (FRAP), and β-carotene bleaching are also employed. Comparing AOA across different studies is complex due to variations in fungal material, solvents, and methodologies. Kozarski et al. [29] evaluated AOA using multiple assays, and reported that F. betulina methanolic extracts exhibited low AOA compared to other fungi. However, they achieved significant inhibition in DPPH (94.44%) at 5 mg/mL, similar to our ethyl acetate extracts (94.04±0.90% for strain 2770).
Compounds inducing ABA and AOA include phenolic acids, triterpenes, and betulinic acid, establishing a link between TPC and AOA [1–3, 24, 29]. In our study, mycelial TPC values were higher and more consistent than those in culture liquids, except for strain 2777, which exhibited the highest TPC in culture liquid. Relative to literature [27-30], F. betulina shows lower TPC in water and methanol extracts of fruiting bodies than other fungal species (e.g., 32.6±2.1 mg GAE/g vs. F. pinicola at 387.7±0.1 mg GAE/g). Our ethyl acetate extracts had even lower TPC (max 8.57±0.18 mg GAE/g). Thus, solvent choice and extraction parameters significantly impact phenolic yields, alongside fungal biosynthesis capacity. Of course, the possibility of biosynthesis by specific fungus should also be considered. The presence of specific phenolic compounds in F. betulina was established. Sułkowska-Ziaja et al. [7] identified syringic acid, gallic acid, 5-hydroxybenzoic acid, and 3,4-dihydrophenylacetic acid in both F. betulina artificially cultivated mycelia and fruiting bodies from nature using methanolic extracts. Kozarski et al. [29] also confirmed various phenolic acids, including protocatechuic and chlorogenic acids, in F. betulina. Also, the presence of phenols, vitamin C, lycopene, and β-carotene in methanolic extracts further highlighted their antioxidant potential.
Our study, using ethyl acetate, addresses the underexplored potential of nonpolar solvents, which are less common (1.88% usage) than water, ethanol, or methanol [35]. Screening solvents is crucial, as solvent choice affects both AOA and TPC. De Bruijn et al. [36] demonstrated that acetone was the most effective for DPPH scavenging in Grifola gargal, whereas water/ethanol extracted higher TPC. Similarly, Atamanchuk and Bisko [37] found that methanol yielded the highest TPC, while ethyl acetate extracts exhibited lower TPC but moderate AOA (41.28–60.83%) in Xylaria polymorpha and X. longipes strains. These differences highlight the influence of fungal strain, extraction method, and habitat on optimizing bioactive compound yields. Certainly, these results should not be generalized to all fungi, as the biosynthesis profile of each species and strain is highly specific, and metabolite extraction outcomes may vary. Nonetheless, some trends can be observed. Polar solvents such as water, methanol, and ethanol were generally preferred. However, ethyl acetate also played a role in isolating bioactive compounds [24, 32]. In our study, the majority of the strong and medium correlations between different parameters were found precisely in mycelial extracts of F. betulina strains. Several positive correlations were observed in mycelial characteristics between TPC and biological activities: ABA and AOA. It could be assumed that mycelial ABA is strongly (against used strains of E. coli and S. aureus) or partially (against K. pneumoniae) related to phenols synthesis. The antibiotic piptamine, mentioned above, is a tertiary amine compound, and therefore has a different origin [32]. The most commonly proposed antimicrobial agents are polyporenic acids, lanostane triterpenes, ergosterol peroxide [1, 2, 24]. This group of compounds belongs to the triterpenes, which are not typically classified as phenolic compounds [38]. However, they possess structural features and functional properties similar to polyphenols. As a result, these triterpenes may also be detected during TPC estimation [39].
In addition, AOA of F. betulina strains was, in some cases, associated with ABA (compared to activity against E. coli, K. pneumoniae, P. aeruginosa, and S. aureus). It is possible that the mechanisms of these biological actions are congruent and caused by similar groups of compounds. For example, polyporenic acid C possesses both ABA and AOA [2].
It is noteworthy that mycelial ABA and AOA negatively correlated with biomass production, suggesting fungal resources prioritize stress-related mechanisms (e.g., antioxidant/antibacterial responses) over biomass synthesis.
Establishing correlations between biological activity and substances accelerates drug discovery, elucidates mechanisms of action, and advances precision medicine. Our study demonstrated a positive correlation between mycelial TPC and biomass quantity. Although TPC is associated with biological activities and theoretically should have an opposite correlation with biomass, this could be explained by the presence of other biologically active groups of compounds in fungal mycelia, different proportions of specific phenols in biomass, and the rate of dissolution in ethyl acetate, used in our investigation.
Culture liquid extracts showed no significant dependence between biological activities (ABA and AOA), between ABA and synthesis of TPC and EP (determined in a study [18]), between AOA and EP, and between TPC and EP. However, fungal polysaccharides may have AOA [40, 41]. These observations could be explained by a different composition of substances in the extracellular metabolites and by the extraction specificity of ethyl acetate. Besides, the GPYB liquid medium used for the cultivation of F. betulina in our study might not be optimal for the production of EP [18].
The strong positive correlation found in our study between AOA and TPC, both in mycelial and culture liquid extracts, could be further evidence of the relationship between these parameters. Perhaps the positive interdependence between ABA, AOA, and TPC could be an important feature of the biological activity and metabolism of the F. betulina. A strong correlation between TPC and AOA of Daedaleopsis confragosa, Fomes fomentarius, F. betulina, Inonotus obliquus, Pycnoporus cinnabarinus, and Trametes versicolor was also shown in a study by Szymański et al. [27]. The same correlation was observed between TPC and AOA, as determined by OH and LPx inhibition assays, for the studied fungi F. betulina, F. pinicola, Ganoderma. applanatum, G. lucidum, and T. versicolor [29].
Strain-specific differences in ABA, AOA, and TPC were consistent across F. betulina and other fungi such as P. ostreatus [17], Hericium coralloides, H. erinaceus [16], F. fomentarius, F. pinicola [25], X. polymorpha, X. longipes [37], and also F. betulina [25].
Overall, our research confirms the biological therapeutic potential of F. betulina strains, highlighting the strain-specific nature of their antibacterial and antioxidant activities, as well as their phenolic content.
CONCLUSION
This study evaluated the antibacterial and antioxidant activities, along with the total phenolic content, of various F. betulina strains. The findings highlight the importance of screening studies in identifying promising producer strains. Among the tested strains, F. betulina 2777 and 2778 stood out. F. betulina 2777 exhibited the highest synthesis of extracellular phenolic compounds, while the mycelial extract of F. betulina 2778 was among the most effective antibacterial agents. Both strains demonstrated significant antioxidant activity in both the mycelium and culture liquid. Based on the data from all F. betulina strains, mycelial extracts generally appeared more advantageous across all biological activities. However, using both polar and nonpolar solvents during extraction could enhance the identification of biologically active compounds and expand the spectrum of synthesized metabolites. Further research is required to identify and characterize biologically active compounds.
Cultivating Birch polypore mushrooms in culture presents opportunities to enhance productivity, optimize incubation conditions, scale up mycelial production, and develop biologically active products for medicinal and veterinary applications.
AUTHORS' CONTRIBUTION
T.Z.: Conducted the research, analyzed and interpreted the data, and wrote the manuscript; V.B.: Reviewed and analyzed the manuscript content, made corrections, and provided critical remarks; M.S.: Analyzed the manuscript content, edited the manuscript, and made corrections; T.K.: Designed and conducted the research, analyzed and interpreted the data, reviewed and analyzed the manuscript content, edited the manuscript, and provided critical remarks.
LIST OF ABBREVIATIONS
ABA | = Evaluation of Antibacterial Activity |
AOA | = Evaluation of Antioxidant Activity |
TPC | = Evaluation of Total Phenolic Content |
MBC | = Minimum bactericidal concentration |
AVAILABILITY OF DATA AND MATERIALS
The authors confirm that the data supporting the findings of this study are available within the article.
FUNDING
This work was financially supported by the projects of the National Academy of Sciences of Ukraine under State Registration Numbers 0121U108000 and 0124U002425.
ACKNOWLEDGEMENTS
We would like to thank Prof. Nina A. Bisko (M.G. Kholodny Institute of Botany of the National Academy of Sciences of Ukraine) for providing Fomitopsis betulina strains from the Mushroom Culture Collection (IBK).