All published articles of this journal are available on ScienceDirect.
Ethanol Production Potential of Sweet Sorghum in North and Central Ukraine
Abstract
Background:
Sweet sorghum (Sorghum saccharatum (L.) Moench) is a unique crop with great potential to serve both the food and energy industries. It is due to the possibility of (bio)ethanol production both from the juice and biomass of this crop. The sorghum stems juice contains sugar in the levels similar to that of sugarcane. Besides, low cultivation requirements for the sweet sorghum make this crop even more attractive for sugar and ethanol production. In terms of technology, sweet sorghum is seen as a transitional feedstock for the first to the second generation bioethanol production. However, effective technological development of the plant cultivation and processing in the Northern and Central Ukraine is restrained by the lack of a collection of sweet sorghum genotypes and adapted varieties for its large-scale cultivation. Additionally, no evaluations of potential (bio)ethanol productivity have been performed for this region, which is important for efficient implementation of novel biofuel-producing technologies and for successful development of a green economy.
Objective:
This research was aimed to create a pool of sweet sorghum genotypes with the involvement of worldwide germplasm, analyze their morphology and breed high-yielding plant lines for the efficient production of liquid biofuels for second-generation bioenergy. Based on that, we also aimed to explore the prospects regarding the efficiency of sweet sorghum cultivation for (bio)ethanol production in the Northern and Central Ukraine.
Methods and Materials:
A valuable gene pool of S. saccharatum (L.) Moench (41 samples) was created; in particular, high-performance genotypes were created for cultivation under the soil-climatic conditions of Ukraine. The bio-morphological features and the yield potential of the plants were determined and the biochemical composition of the phyto-raw materials was determined in different periods of vegetation, in particular, during the technical ripeness of the above-ground mass of plants. The more productive forms and varieties of sugar sorghum in terms of yield, dry matter content, sugar, and energy value of biomass during flowering and waxy ripeness are highlighted. The technological properties of plant biomass for the production of alternative liquid fuels (in particular, bioethanol) have been analyzed. Importantly, optimal cultivation conditions have been elaborated for the newly created sweet sorghum genotypes, and their productivity has also been evaluated. Moreover, for the first time, a detailed study on potential ethanol yield has been conducted.
Results:
Sweet sorghum has considerable potential in Ukraine as a new sugar-producing energy crop. The germplasm collection of this crop has been created (41 accessions), including introduced and acclimated genotypes and newly bred lines and varieties. The biological performance of sorghum in Ukraine and plant morphology have been analyzed. The most promising genotypes were used for breeding of new high-productive sweet sorghum varieties. The potential (bio)ethanol yield for different sugar feedstocks (juice, grain bagasse) can reach up to 11423 L/ha in total from juice, grain and bagasse.
Conclusion:
The estimated values of ethanol productivity are comparable to the results of other similar investigations. In conclusion, a high performance of sweet sorghum in Ukraine can be suggested.
1. INTRODUCTION
One of the negative consequences of global climate change are prolonged droughts during the season of plant cultivation. To address this challenge, interest in drought-tolerant, low-input and high-yield crops is rapidly growing [1, 2]. Such promising crop candidates are plants of Sorghum genus, which are extensively used for feed, food and energy purposes. Such valuable properties of Sorghum species such as their high genetic potential, the ability to use efficiently agro-climatic resources, as well as high heat- and drought-tolerance of these crops are extremely attractive. The largest Sorghum sowing area is allocated in the countries of North and South America, Asia (China, India, Central Asian countries) and Africa. Globally, it occupies more than 40 mln ha [2-6].
The homeland of Sorghum is North-East Africa, in particular Ethiopia and Sudan, where most of its wild species and cultural forms are currently found. Historically, Sorghum crops have been cultivated since the IV-III centuries BC [7]. The high productivity of sorghum and relatively low requirements of the soil quality make this crop extremely attractive for cultivation for both forage and bioenergetic purposes [8]. It can be grown on soils unsuitable for food or feed production [9]. It also features a number of economically desirable properties such as wide adaptability, high biomass productivity, resistance to pests and plant diseases, drought tolerance, etc [10].
In Europe, a special program to increase sorghum production – Sorghum ID (International Development Sorghum) - was implemented (https://www.sorghum-id.com/ en/overview/). One of the initiators of this program was the French Federation of Corn and Sorghum Seed Producers (FNPSMS). To promote this crop, European Sorghum – Real Potential Congress was held in Bucharest in 2016, bringing together over 250 key players on this market. Since 2017, a three-year promotion plan for Sorghum with high potential has been implemented. 7 European countries – France, Italy, Spain, Romania, Bulgaria, Ukraine and Russia - have great prospects for using Sorghum. Today, the research on improving the technology for producing bioethanol from sorghum stem juice is being conducted globally [11-15].
Most of the sorghum crops grown in the world are used for sugar and ethanol production. Sweet sorghum (S. saccharatum) includes a large number of varieties, which are characterized (in contrast to the S. bicolor and S. technicum) by high sugar content, from 10 to 20% and more, in the stalk juice. The stems usually contain at least 50% (by weight) of juice [8]. In terms of sugar content, the sweet sorghum juice is not inferior to sugarcane but, in addition to sucrose, it contains fructose, glucose and soluble starch [16-19].
Since recently, sweet sorghum has been considered to be an ideal energy crop for the production of first- and second-generation bioethanol. The sugar content of the Sorghum stems and their juiciness are the main factors that define the efficiency of bioethanol production. The possibility to control these features at the genetic level has recently been reported [20]. Besides the high amount of sugar in its juice, sorghum showcases a high productivity of grain, which is rich in starch [21]. Raw biomass of this crop or bagasse has been used recently as a promising feedstock for biogas production [13, 22, 23]. Thus, bioethanol from Sorghum can be produced at a conventional alcohol production plant [24].
As a potential bioenergy crop, sweet sorghum is of great interest in different countries around the world. In 2009, the European Union initiated an international project titled “SWEETFUEL” aimed to improve the yield capacity of sorghum cultivars. Apart from the European countries, Brazil, Mexico, India, and South Africa also participated as partners in this consortium. As an outcome of this project, several recommendations have been made that include designing ethanol plants for the full utilization of leaves as well as the surplus bagasse [3]. In 2009, a joint venture financing for EPEC Biofuels (FL, USA) was set up to initiate the development of systems for ethanol production from sweet sorghum in Southern USA [1].
This crop has also been included in the list of the best sources of liquid biofuels in terms of the development of renewable energy in China [25]. The first successful commercial plant with sweet sorghum as a feedstock was approved and established in Inner Mongolia in 2012 [15]. Additionally, the efficiency of sorghum cultivation on marginal lands for ethanol production was shown recently for Inner Mongolia [26].
In Ukraine, sweet sorghum is considered a highly valuable, bioenergetic, technical, fodder, and phytomeliorative crop. The biomass of sweet sorghum is similar to corn in terms of chemical composition, but its yield is much higher and usually reaches up to 40-80 t/ha. Sorghum stems (stalks) comprise over 75% of the biomass yield. Sweet sorghum also produces grain, which is rich in starch (68-73%) and protein (11-15%) [27-31]. However, seed production of this crop is not conducted on an industrial basis in Ukraine. Unfortunately, the cultivation of this crop is limited to the Northern and Central Ukraine due to the lack of adapted high-yielding genotypes, suitable for cultivation under the Forest-Steppe climatic conditions for further ethanol production.
As a result of a long-term investigation, a unique collection of sweet sorghum germplasm has been created in M.M. Gryshko National Botanical Garden (NBG) of the National Academy of Sciences of Ukraine. Moreover, a pool of valuable genotypes and varieties was bred at the Department of Cultural Flora of NBG, which possess a high adaptational ability to be cultivated in northern regions of Ukraine as well as provide high productivity, in terms of yields and potential ethanol output.
Therefore, in this study, we have investigated the morphology, adaptability and yield traits of representatives of sweet sorghum NBG germplasm collection, as well as determined optimal sowing/fertilization conditions for the cultivation of sweet sorghum with the aim to maximize plant productivity for increasing potential ethanol yields. Finally, total bioethanol yields (from juice, grain and bagasse) have been estimated to evaluate the sweet sorghum biofuel productivity potential.
Deposited at NBG | Acclimated Genotypes | Created Breeding Lines | Registered Cultivars in Ukraine |
---|---|---|---|
AMBR-1 | ETSSTSFKIA-2-9 | ETSSTSF-1* | Botanichnyi* |
AMBR-1-1 | ETSSTSFM-5 | ETSSTSF-1-2 | Energodar |
AMBR-2 | ETSSTSFM-5-1 | ETSSTSF-2* | Kynelske-9 |
AMBR-2-1 | ETSSTSFPSH-7 | ETSSTSF-2-1 | Medove |
AMBR-4 | ETSSTSFRR-1 | ETSSTSF-2-6 | Pamiati Shepelia |
AMBR-5 | ETSSTSFRR-2-9 | ETSSTSF-2-7 | Progres |
AMBR-6 | ETSSTSFRT-1 | ETSSTSF-3* | Yantar |
RUSBR-2 | ETSSTSFTK-1 | ETSSTSF-4* | Bilotsukrova |
RBSBR-4 | ETSSTSFVS-3 | ETSSTSF-5* | - |
RUSBR-4-1 | - | ETSSTSF-6* | - |
SAMS-1 | - | ETSSTSF-7* | - |
- | - | ETSSTSF-8 | - |
- | - | ETSSTSF-9 | - |
2. MATERIALS AND METHODS
2.1. Plant Material
A total of 41 Sorghum saccharatum (syn = S. bicolor var. saccharatum) accessions, deposited at the Bioenergetics crops collection in NBG, were used in this study (Table 1); 8 of which were used for additional investigation of bioethanol productivity. Besides, other species of Sorghum genus were also investigated, including one genotype of S. technicum (syn = S. bicolor var. technicum), one S. vulgare (syn = S. bicolor var. vulgare), one S. bicolor, two breeding lines (Breeding line 1 and Breeding line 3) of S. durra (syn = S. bicolor var. durra) and one hybrid line S. durra x saccharatum (syn = S. bicolor var. durra x saccharatum).
2.2. Conditions of Plant Cultivation and Experimental Design
The 34 accessions, used for morphometric measurements (listed in Table 1, excluding ETSSTSF-1; -3; -4; -5; -6; -7, cv. Bilotsukrova), as well as genotypes, used for comparison of different Sorghum species productivity, were grown on experimental plots at the M.M. Hryshko National Botanical Garden of the National Academy of Sciences of Ukraine (50°32’N, 30°33’E). The plot size was 4 m long and 4 rows wide, while the spacing between the rows was 70 cm and 10 cm between the plants. Each genotype was cultivated on a separate testing plot (n=4). The soil was classified as alfisol (рН 6.1-6.8, measured at 1:2 soil to water ratio). The average air temperature and precipitation in this area were +7.6oC and 550-560 mm per year. Fertilizer grade 16–16–16 (N–P–K) at the rate of 60 kg/ha was applied to the test plots twice during the planting and 30 days after the planting. The scheme of plot allocation was randomized complete block design (RCBD) for 4 replicates.
The field research was conducted in the Agricultural Experimental Production Station “Hlevakha” of the Institute of Plant Physiology and Genetics of the National Academy of Sciences of Ukraine (50015’N, 30017’E). The soils there were also classified as alfisol (рН 6.0-6.7, measured at 1:2 soil to water ratio), with humus content of 2.5-2.6%. The average air temperature during the growing season (March-November) was +14.6 °C, while the annual average was +7.7 °C. Rainfall during the growing season varied from 432.3 mm to 623.8 mm. All variants of the experiment were cultivated on separate testing plots (n=4).
Breeding lines (ETSSTSF-1; -2; -3; -4; -5; -6; -7) were cultivated to identify their morphological differences and to establish their productivity rates, which were further used for ethanol productivity estimation. Plot sizes and rows/plants spacing were the same, as described above. Fertilizer 16–16–16 (N–P–K) was also applied at the rate of 35 kg/ha to the testing plots during the planting and then again, 30 days after the planting.
Cultivar Botanichnyi was used in a series of experiments, focused on thefertilization effect on plant productivity. In the control variant, no soil fertilization was applied. Bacterial inoculums - biopolycide (spores of Paenibacillus polymyxa, strain 6M) and phosphobacterin (mass spores of Bacillus megaterium), used for plant pre-treatment, were obtained from the Institute of Agroecology of National Academy of Agrarian Sciences of Ukraine. Seeds were pre-treated with biopolycide (0.5-1x1012 of spores per 1 mL) at the rate of 15 mL per 1 kg of seeds. The inoculum was diluted with water to 1% solution and applied on the day of sowing. Phosphobacterin (1.0-1.5x109 of spores per 1 mL) was applied also prior to sowing at the rate of 2 mL per 1 kg of seeds (inoculum was diluted with water to 0.2% solution). For a combined variant of pre-treatment, inocula were taken at the same rate per kg of seeds and were diluted in 1.5 L of water. For 16–16–16 (N–P–K) soil supplementation variant, the fertilizer (at the rate of 135 kg/ha) was applied to plots on the day of sowing and after 30 days from the planting date.
The effect of different rows/plants spacing was tested also using cv. Botanichnyi. The plants were sown in different manner (spacing between rows x plants): 70x30, 70x20, 70x10, 45x30, 45x20, 45x10, 15x30, 15x20, 15x10 cm. Prior to sowing, the seeds were treated with biopolycide, similarly to the way described above. In all mentioned testings, the scheme of plot allocation was also RCBD for 4 replicates.
2.3. Morphological Data Collection and Plant Productivity
Morphometric data was collected at two main periods: the flowering phase (when more than 50% of plants started flowering and shed pollen) and the seed ripening phase (30 days after the flowering) from 10 randomly chosen intact plants in each plot. The same procedure was repeated during the harvest (30 days after flowering of each cultivar starting approximately in mid-September). Plant height, stalk diameter, leaves and inflorescence size were recorded from 10 randomly chosen plants in each plot. Plant height was measured as a distance from panicle (or inflorescence) tip to the ground. Stem (stalk) diameter was measured at three different positions using a caliper. Plants were harvested manually. To do that, stems were cut at the ground level, and then panicles and leaves were removed. The weight of each plant organ was recorded individually for 10 random plants per plot. The total weight of stripped stalks and total biomass weight were recorded for each plot. Additionally, the weight of panicle (with seeds), the weight of seeds from one panicle, the weight of 1000 seeds and the total weight of seeds collected from each plot were recorded.
The stalks of sweet sorghum were crushed to extract the juice using a roller crusher. Extracted juice was then immediately stored at −20°C for further analysis. Specific gravity was calculated as juice weight divided by the volume of weighted juice. Total soluble solids of the juice were determined using a hand-held refractometer to measure Brix (sugar content in juice) immediately after squeezing of the juice.
2.4. Biochemical Analyses
Samples for biochemical analysis were collected at the above specified sweet sorghum growth stages. Dry matter content was measured for collected stalks. Stems were chopped, milled and immediately weighted. After that, the plant material was dried in a heat-oven at 105°C for 3 days. After drying to the constant mass, the resulting weight was recorded and used to calculate dry matter (as a relation of dry weight to fresh weight, expressed in %).
The total content of reduced sugars in stalks was evaluated by Bertrand’s method (with slight modification) based on the reducing effect of sugar of alkaline solution on tartrate complex with cupric ion. The cuprous oxide formed was dissolved in a warm acid solution of ferric alum. The ferric alum was reduced to FeSO4, which was titrated against standardized KMnO4; Cu equivalence was correlated with the table to get the amount of reducing sugar. This method was based on the alkaline solution of the tartrate complex of cupric ion [32]. Data were expressed in % of the weight of fresh stalks.
The amount of energy in the samples was determined with a calorimeter ІКА С 200 (IKA®-Werke GmbH & Co. KG, Germany). Apart from the plant material, collected during field growing, sorghum bagasse was also used to determine the energy value of biomass after the juice pressing (that was done only for genotypes AMBR-2, AMBR-5, cv. Botanichnyi, cv. Bilotsukrova, cv. Medove and cv. Yantar). Measuring of the caloric content was performed as follows: 0.1-0.2 g of dried plant material (measured exactly with the weight figure uploaded onto the calorimeter) was brought in oxygen bomb (decomposition vessel IKA C 5010/5012) and put in distilled water in the calorimeter. The procedure lasted for 13-15 min. Benzoic acid 723 was used for the standardization of the process. Data were expressed in kcal/kg.
2.5. Estimation of Potential Ethanol Yield
Several equations were used to estimate potential ethanol yield from the investigated sweet sorghum genotypes. Prior to calculating the ethanol output, some yield parameters were also estimated, based on the data of the above-described measurements. To determine the approximate yield of sugars (t/ha), the equation, described by Wortmann et al. [33], was used:
![]() |
(1) |
where 0.75 is the production index, introduced to account for potential losses at a particular technological stage.
Since some of the equations required the input of another type of crop productivity data, we used the following two equations, based on the previous ones (1):
![]() |
(2) |
![]() |
(3) |
where producing index (0.866 in both equations (2) and (3)) is derived from 0.75 production coefficient .
We also used three other different equations to estimate the ethanol output more accurately. The first one was successfully used to determine the influence of various crop cultivation factors on ethanol production [34] and originally described by Lipinsky [35]:
![]() |
(4) |
This equation (4) is based on stalk (stems) yield (t/ha) and requires measurements of soluble solids or sugars content, expressed in % w/w of the stems weight. The equation is based on the sugar to ethanol conversion rate (0.65 L of ethanol per 1 kg of sugar) and assumes 85% (0.85 index) of the production efficiency.
Two additional estimation methods were chosen since their accuracy was successfully tested against the real ethanol production experiments [36]. The first one was originally described by Smith et al. [37]:
![]() |
(5) |
This equation (5) requires sugar yield (t/ha) data that we derived by the method, described above in (1). The ethanol/sugar conversion of 0.665 (L/kg) was taken as an average of 0.648 (L/kg) of hexose conversion rate and 0.682 (L/kg) of sucrose conversion rate [36]. The same average conversion rate was also used in the previous estimation method (4). Also, this (5) equation was found to be more accurate when assuming the production efficiency of 80% (0.8 coefficient) [36].
The following equation was originally proposed by Somani et al. in 2003 [38]:
![]() |
(6) |
where SG – specific gravity of juice.
This equation (6) is based on juice productivity (t/ha) that we estimated with the formula (2). The ethanol/sugar conversion rate of 0.53 (L/ha) was used as in equation (5) but with 0.8 production coefficient.
To estimate the potential yield of ethanol, the calculation was done using our proposed equation:
![]() |
(7) |
where sorghum grain yield is expressed in kg/ha, taking into account the content of water-soluble starch in % (w/w).
A reference value of soluble starch content in equation (7) was used as 67.75% w/w, which was a mean rate, suggested in a respective sorghum collection study [39]. The ratio of 0.9 kg glucose per 1kg of starch reflects the efficiency of enzymatic hydrolysis of sorghum starch. This value is also a mean, based on two respective studies on sorghum grain starch hydrolysis and fermentation [40, 41]. Hexose to ethanol conversion of 0.648 (L/kg) was used, as well as an 80% production rate.
A theoretical ethanol yield from sweet sorghum bagasse (SSB) was calculated on the basis of existing SSB-to-bioethanol conversion rates. Therefore, the calculation was done as follows:
![]() |
(8) |
where kg of dry SSB is an amount of stems dry matter yield with a production efficiency coefficient of 0.85.
As reference values of SSB (8) to bioethanol conversion rates determined for technologies with different biomass pre-treatment methods: 163 g EthOH/kg SSB for SO2-steam explosion and following S. cerevisiae fermentation were used [42, 43], 184 g/kg for acidic pre-treatment and fermentation with genetically engineered S. cerevisiae [44]; 275 g/kg for steam explosion with H3PO4 impregnation followed by E. coli SL100 fermentation, were observed [45]. Conversion of bioethanol weight to volume was done at the 0.789 cm3/g density (value for 99.5% bioethanol) [46]. In the final evaluation, a mean value of all SSB bioethanol calculation variants was used.
2.6. Statistical Analysis
All statistical processing of the obtained data was conducted using OriginPro 9.1 software. Deviations of all means were calculated as a standard deviation (SD). To identify the significance of differences in different morphological and productivity parameters between the studied genotypes, one-way ANOVA was used, which included the calculation of Fisher’s least significant differences (LSDs). The LSDs were used to identify homogeneous groups for values of particular morphological or productivity parameters (at p<0.05 significance level). In some cases, SD of means has not been denoted in tables (where it was considered not important for data representation), since one-way ANOVA was applied to all datasets.
Two-way ANOVA was conducted to identify the significance of influence of factor pairs (genotype and sugars content (Brix%) in juice) on the energy value change of sweet sorghum biomass before and after juice pressing (δkcal/kg). For verification of the significance of differences, LSDs were also calculated (at p<0.05 significance level).
Pearson correlation analysis was conducted using the same software and was applied to the data of morphology measurements of 34 accessions (mentioned above) during two plant growth stages (flowering and seed ripening). Correlations were considered significant at p<0.05 level.
3. RESULTS AND DISCUSSION
3.1. Phenotyping of Sweet Sorghum Genotypes
A morphology screening study of the sweet sorghum diversity was conducted using 34 accessions from the collection of a total of 41, deposited at the Department of Cultural Flora of NBG. Specimens from Europe, Asia, Africa, and America were represented in the gene pool of forms and varieties of S. saccharatum (Table 1, first column). As a result of many years of plant introduction studies, we have been able to obtain seed-reproducing lines of all these genotypes. A gene pool of fully acclimated sweet sorghum lines has been created (Table 1, second column), which in some cases required crossing with local genotypes. This allowed us to create several breeding lines (Table 1, third column) with high adaptability to cultivation conditions and optimal productivity. Individual genotypes were further used as a basis for the creation of new cultivars, including one successfully registered – cv. Botanichniy.
Sweet sorghum is a new high-performance bioenergy crop in Northern and Central Ukraine. It is an annual plant, which passes all stages of organogenesis in one growing season under local climate conditions. The flowering of plants takes place in July-August, while seed ripening occurs from the second half of August to the end of September. The growing season lasts from 120 to 180 days depending on the genotype. Sweet sorghum is a thermophilic plant. Its seeds can germinate at a soil temperature of 10-12°C, while seedlings do not withstand temperatures below 0°C. It can grow and develop well at a temperature of 30-36 °C, easily withstanding high temperatures up to 40°C. Besides, sweet sorghum is a drought-resistant plant, therefore it can easily tolerate precipitation level [47], typical for temperate climate zone (including Forest-Steppe zone). Especially interesting from an environmental point of view is the cultivation of S. saccharatum at an industrial scale for the production of biomass and sugars on the soils contaminated with heavy metals [48].
The conducted screening of sweet sorghum germplasm allowed determining plant height (cm), stems, leaves and panicles weight (g), the total weight of the aboveground mass per plant as well as dry matter content (%) and biomass energy value (kcal/kg). Sorghum plants are able to grow more than 300 cm in height forming long-lanceolate leaves. The root system is fibrous, highly developed, which grows 150-200 cm in depth. Additionally, aerial roots are also formed. Depending on their height, the plants can be separated into three groups: first – 151-200 cm; second - 201-250 cm; third – 251 cm and above. Previously, for the creation of breeding lines, genotypes from the third group were used. During field trials, the highest plants were found in the plots of ETSSTSF-2 (breeding line), accessions AMBR-1-1, AMBR-3, ETSSTSFM-5 and cultivars Prohres, Kinelske-9 and Enerhodar.
Correlational analysis was conducted based on the phenotyping data that resulted from measurements at a flowering phase (Fig. 1) and at a seed ripening phase (Fig. 2). No significant correlations have been established between plant height and other parameters, including the aboveground mass. This may allow to suggest that, probably, stem diameter makes a greater contribution to the biomass accumulation. According to our results, the highest total biomass (aboveground mass) productivity was observed for accessions AMBR-1, AMBR-3, ETSSTSFM-5-1, ETSSTSFRR-2-9; breeding lines ETSSTSF-2, ETSSTSF-2-1, ETSSTSF-9; and cultivars Botanichnyi and Enerhodar. The strongest correlation was observed between total biomass weight and stems weight on both growth stages r=0.993-0.994 at p<0.01. Thus, the biggest biomass weight comes from the stems (approximately 71% at both stages), regardless of the genotype or general productivity of a particular line.
It is worth noting that significant (at p<0.01) correlations between stem and leaves weight were observed at both stages of sweet sorghum growth (r=0.922 at flowering stage and r=0.698 during seed ripening). At the same time, correlation of inflorescence weight with either leaves (r=0.471) or stems (r=0.395) weight was significant at p<0.05, but weak; while during seed ripening, these correlations were much stronger (r=0.624 with leaves and r=0.875 with stems) at low p-value (at p<0.01). Similar patterns of growth parameters were also observed in other countries [5, 22].
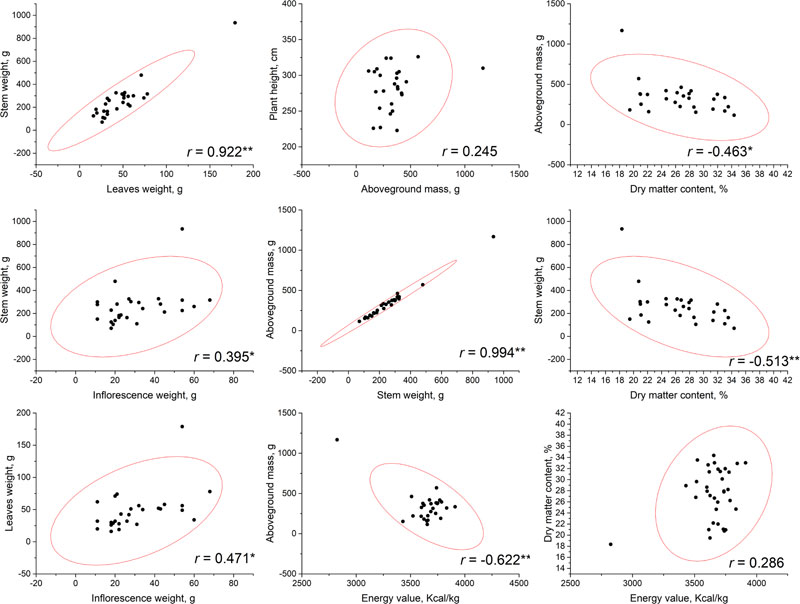
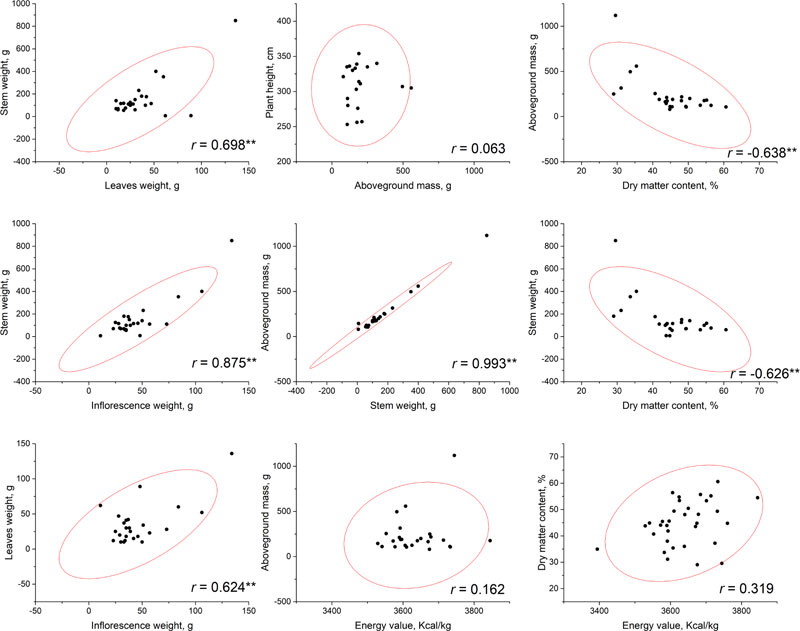
Sweet Sorghum Genotypes | Mass of the Panicle, g | Mass of the Seeds Per Panicle, g | Weight of Seeds in Panicle, % | Mass of 1000 Seeds, g |
---|---|---|---|---|
ETSSTSF-1 | 45.90±6.61a | 37.90±6.02a | 82.57a | 23.09±0.07a |
ETSSTSF-2 | 31.20±3.38b | 23.22±2.34b | 74.42b | 19.94±0.16b |
ETSSTSF-3 | 30.60±3.05b | 24.88±2.09b | 81.31a | 19.11±0.56b |
ETSSTSF-4 | 16.40±1.25c | 13.22±0.78c | 80.61a | 18.20±0.34b |
ETSSTSF-5 | 27.30±2.58b | 22.0±2.07b | 80.59a | 19.74±0.11b |
ETSSTSF-6 | 29.22±4.05b | 20.29±2.14b | 69.44c | 20.07±0.20b |
ETSSTSF-7 | 31.30±3.80b | 26.0±3.24b | 83.07a | 18.28±1.51b |
Mean | 30.27±8.81 | 23.93±7.52 | 78.86±4.95 | 19.78±1.65 |
The energy value of dry biomass ranged from 2824.5 to 3911 kcal/kg during flowering and from 3394.2 to 3745.1 kcal/kg by the end of the seed ripening period. Interestingly, while flowering, a significant (at p<0.01) negative correlation (r=-0.622) was identified between biomass energy value and the aboveground mass of the plant, while no significant correlation between these parameters was observed. Additionally, no significant correlation was found between energy value and dry matter content during both growth stages.
Dry matter content of biomass, obviously, increased from flowering stage to the end of vegetation stage of plants. At the flowering stage, dry matter content varied from 18.34 to 34.36% and after the end of seeds ripening, it reached from 29.04-60.63%. The highest values were detected within breeding lines ETSSTSF-1-2 (60.63%), ETSSTSF-2-6 (56.41%); and cultivars Progres (55.72%) and Yantar (54.49%). Such high moisture loss could potentially negatively impact the juice output, thus reducing ethanol production efficiency. Cv. Botanichniy contained 44.07% of dry matter, which was very close to the mean value within the analyzed genotypes pool, i.e. 44.8%. On the other hand, these measurements were performed after the period of the supposed harvest of stalks for juice production; therefore, moisture content was expected to be higher at that time. Nevertheless, significant but not very strong negative correlations were observed between dry matter content and the above-ground mass weight (at flowering r =-0.463, p<0.05 and after seeds ripening – r=-0.638, p<0.01). More significant (both at p<0.01), but not stronger, negative correlations were observed between dry matter content and stalk weight, r=-0.513 at flowering and r=-0.626 during seed ripening. In general, loss of moisture by the plants during their vegetation (after flowering) led to a decrease in the total weight of stalks and biomass in general.
Important productivity traits for many crops are quality and quantity of seeds produced per plant. In Table 2, main parameters indicating seed productivity of several sweet sorghum breeding lines are provided. During the field trial, it was recorded that the length of the panicle usually reached 60-70 cm. Seeds of sorghum have an elongated form and differ in color, while the weight of 1000 seeds varies between 18.2 and 23.09 g. Typically, most of the weight of sorghum panicle is due to seeds, which comprise about 78.86% of the total panicle weight. The largest panicle weight (45.9 g) was found in the breeding line ETSSTSF-1, while the smallest (16.4 g) in ETSSTSF-4, respectively. ETSSTSF-1 delivered the highest yield of seeds per panicle (37.9 g, which is 82.57% of the total weight) and the highest weight of 1000 seeds was 23.09 g. Other breeding lines produced smaller panicles of about 30.37 g, containing on average 23.93 g of seeds.
3.2. Sorghum Species and their Bioethanol Producing Potential
Apart from S. saccharatum, there is a number of species, which could also potentially be used as biofuel (ethanol) feedstocks. Most of them feature either comparable or lower biomass productivity than sweet sorghum (Table 3). However, all Sorghum species included in this comparison are now considered to be subspecies or varieties of S. bicolor (The Plant List, http://www.theplantlist.org/tpl1.1/record/kew-443283). Therefore, there is a possibility to obtain ‘interspecies’ crosses, which was demonstrated in the example of the hybrid line (S. durra x saccharatum), obtained out of grain sorghum and sweet sorghum. On the other hand, data of molecular genetic fingerprinting of different Sorghum species suggest that S. bicolor, S. technicum and S. saccharatum are the most closely related; however, their representatives are sufficiently distinctive to form separate groups (clades) for each of these species [49].
The lowest biomass productivity was recorded within S. vulgare – 38.13 t/ha, while the highest one was found for sweet sorghum (cv. Botanichniy) – 82.74 t/ha and S. technicum – 79.86 t/ha. The hybrid plant possessed lower biomass accumulation – 72.96 t/ha. Similarly, as reported above, most of the biomass weight was observed in the stalks (approximately about 71%, regardless of the species). The content of sugars in stalk juice was significantly lower within all sorghum species (12.87-13.94%), except sweet sorghum (19.66-21.41%) and S. durra x saccharatum hybrid (19.1%).
Additionally, potential ethanol yield was estimated for each of the analysed species on the basis of the obtained field trial data (Table 3). The estimated EthOH output was not significantly different for S. bicolor, S. durra and S. technicum and was in the range of 1817.1-1986.9 L/ha. At the same time, sweet sorghum possessed significantly higher values of 3234.9-3538.8 L/ha, while the S. durra x saccharatum hybrid showed an intermediate value of 2635.5 L/ha, comparable to parental species. Other researchers reported similar low ethanol yields from forage sorghum of up to 1051 L/ha only [50]. Such low performance of other sorghums, in terms of ethanol yields, was expected, since these species (or subspecies) were not specifically bred for the accumulation of high levels of sugars.
Estimated grain yield values have also been identified for some of the investigated species (Table 4a). The highest productivity was received by Breeding form 3 of S. durra – 6.3 t/ha and sweet sorghum cv. Botanichniy – 6.4 t/ha, S. vulgare and Breeding form 1 produced 5.5 t/ha each. Concerning the conversion of grain to ethanol, the potential alcohol output can be increased by additional 1738.5-2023 L/ha. Regarding the general possibility of ethanol production, sweet sorghum holds the leading position compared to other investigated species/subspecies. Otherwise, S. durra x saccharatum hybrid line could be of interest for further breeding improvement.
As reviewed by Anglani, the nutritive value of sorghum is lower than that of other cereals due to the lower content of some amino acids and the presence of antinutritional tannins [51]. For this reason, Sorghum is not very good for breadmaking flour [51]. On the other hand, this opens possibilities for industrial utilization of its grain.
Species | Total Biomass Yield, t/ha | Sugar Content in Juice, % | Estimated Bioethanol Output, L/ha |
---|---|---|---|
S. technicum | 79.86a | 12.87c | 1986.9±393.6c |
S. vulgare | 38.18c | 12.93c | 954.3±188.6d |
S. bicolor | 68.14b | 13.94c | 1847.8±353.1c |
S. durra (Breeding form 3) | 69.49b | 13.48c | 1817.1±352.4c |
S. durra x saccharatum (hybrid) | 72.96b | 19.1b | 2635.5±451.9b |
S. saccharatum (ETSSTSF-1) | 76.4ab | 19.66ab | 3234.9±550.4a |
S. saccharatum (cv. Botanichnyi) | 82.74a | 21.41a | 3538.8±589.8a |
Species | Grain yield, t/ha | Ethanol yield, L/ha |
---|---|---|
S. saccharatum (cv. Botanichnyi) | 6.4a | 2023.0a |
S. durra (Breeding form 1) | 5.5b | 1738.5b |
S. durra (Breeding form 3) | 6.3a | 1991.4a |
S. vulgare | 5.5b | 1738.5b |
3.3. Approaches to Enhance Sorghum Productivity for Increased Ethanol Output
3.3.1. Breeding of New Sweet Sorghum Forms
The pool of breeding lines was created at NBG on the basis of acclimated sweet sorghum lines (Table 1). The most productive ones were selected for further research. Morphological characteristics of these breeding lines are provided in Table 4b. No significant difference was indicated for the height parameter. Although its mean value was 252.94 cm, lines ETSSTSF-1 and ETSSTSF-3 were slightly higher (271.2 and 267.6 cm, respectively) than other lines. Inflorescence (panicle) length varied from 22.2 cm (ETSSTSF-4) to 36.1 cm (ETSSTSF-1). In general, a bigger panicle can produce more seeds, but from the point of view of juice production, it is more desirable to have a higher plant with a small panicle to increase the size of the stalk. Another aspect that can impact juice yield is the stem diameter, which was found to be approximately 11.31 mm. The differences of this parameter were not significant for almost all investigated breeding lines, except the ETSSTSF-6. The highest number of internodes was detected in plants of ETSSTSF-4, while the difference with others was not significant. The average leaf length was 70.39 cm.
As the next step, ethanol productivity-related traits were evaluated (Table 5). The highest biomass productivity values were demonstrated by ETSSTSF-4 - 109.22 t/ha of total biomass, ETSSTSF-3 and ETSSTSF-5 – at 94.57-95.46 t/ha and ETSSTSF-1 – 76.37 t/ha, respectively. The stalk yield was lower and varied in the range of 29.0-77.5 t/ha. The highest dry matter content (and, therefore, the lowest juice content) was received within ETSSTSF-1 at 48% (dry matter content) and ETSSTSF-3 and ETSSTSF-6, both at 45%. The maximum juice yield reached 44.5 t/ha, while the highest sugar content was detected within genotypes ETSSTSF-1 (19.8%) and ETSSTSF-4 (16.3%). According to Adinurani et al. [32], the fresh weight of sorghum was 81.27–210 t/ha, the number of internodes was 7.11–11.77, the measured stem height was 181.29–301.28 cm, and the stem diameter was in the range of 1.27–1.40 cm.
Finally, the potential ethanol yield per hectare from each of the investigated breeding lines has been suggested. The estimation has been worked out using three different equations, according to the procedure described above. The mean value of three separate calculation methods of ethanol yield is presented in Table 4a. According to our estimation, the highest potential ethanol output can reach up to 3676.4 L/ha out of the juice of ETSSTSF-4. Lower ethanol amount could be yielded from ETSSTSF-1, ETSSTSF-3, ETSSTSF-5 – in the range of 2486.5-2698 L/ha. Similar calculations for the other genotypes have shown significantly lower levels of ethanol output. The mentioned genotypes that showed highest productivity and high sugar content were used as the basis for breeding of cv. Botanichniy (Fig. 3). The productivity evaluation of cv. Botanichniy is presented in the following sections.
Genotype | Height, cm | Inflorescence Size, cm | Stem Diameter, mm | Number of Internodes | Leaf Length, cm |
---|---|---|---|---|---|
ETSSTSF-1 | 271.2±15.85a | 36.1±7.82a | 12.7±2.11a | 7.0±0.67b | 67.8±8.72a |
ETSSTSF-2 | 246.4±18.56a | 29.6±2.07a | 11.1±4.07a | 8.0±0.94b | 77.2±6.83a |
ETSSTSF-3 | 267.6±37.55a | 31.6±3.63a | 13.4±2.99a | 8.4±0.84ab | 71.2±7.97a |
ETSSTSF-4 | 244.2±13.31a | 22.2±3.08b | 12.5±0.53a | 9.5±0.53a | 72.2±2.82a |
ETSSTSF-5 | 245.6±15.18a | 24.0±2.71b | 10.6±2.50a | 8.0±0.67b | 59.6±12.38ab |
ETSSTSF-6 | 246.4±14.09a | 30.2±5.92ab | 7.6±1.43b | 6.9±0.74b | 66.7±4.52a |
ETSSTSF-7 | 249.2±14.47a | 29.8±7.10ab | 11.3±3.56a | 6.8±0.42b | 78.0±7.69a |
Mean | 252.94±19.97 | 29.07±6.14 | 11.31±2.9 | 7.8±0.93 | 70.39±8.91 |
Genotype | Biomass Yield, t/ha | Total Dry Matter Yield, t/ha | Sugar Content in Juice, % | Estimated Ethanol Yield, L/ha |
---|---|---|---|---|
ETSSTSF-1 | 76.37c | 36.86b | 19.8a | 2698.0b |
ETSSTSF-2 | 53.28d | 20.35c | 14.8c | 1699.4c |
ETSSTSF-3 | 95.46b | 43.18a | 15.1c | 2486.5b |
ETSSTSF-4 | 109.22a | 35.76b | 16.3b | 3676.4a |
ETSSTSF-5 | 94.57b | 35.99b | 13.9d | 2547.1b |
ETSSTSF-6 | 40.85e | 18.32c | 15.1c | 1264.6d |
ETSSTSF-7 | 47.51de | 19.25c | 13.1d | 1312.3d |
Mean | 73.89±25.8 | 29.96±9.85 | 15.4±2.1 | 2240.6±869.1 |
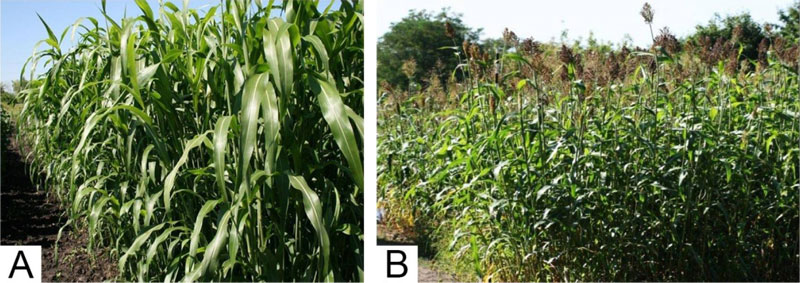
3.3.2. Row Spacing
In the next stage of our investigation, we have tested different sowing variants of sweet sorghum. These experiments were done using cv. Botanichniy mentioned above. The following row spacing variants were tested: 70x30, 70x20, 70x10, 45x30, 45x20, 45x10, 15x30, 15x20, 15x10 cm; the plants were pre-treated with biopolycide in all cases. Morphological parameters of plants were measured for each sowing variant (Table 6) at the harvest time. The results showed that the main indicators of plant growth (plant height, number of internodes, number of leaves and their size, stem diameter) were significantly dependent on the sowing method.
The maximal height value was identified within 70x20, 45x20, 45x10 sowing variants as 386 cm, 394 cm and 381 cm, respectively. Smaller spacing led to lower plant height, up to 212 cm for 15x10 pattern, except for 15x20 variant, where the plant height reached 276 cm. The shorter row spacing would lead to a smaller stem diameter. The thickest stems were identified in plants, sown in 70x20 and 70x10 cm spacing distances and reached 16 mm. Other parameters also showed the same trend of reduction in the number of internodes, the number of leaves and leaf length with the shorter distances between rows and plants. It is of interest to note that diminishing these parameters in 70x30-10 and 45x30-10 sowing options was statistically almost insignificant, except for all 15x30-10 cm variants.
Additionally, biomass productivity and potential ethanol yield have been estimated for each of the sowing variants (Table 7). As a result, the highest yields were received from the plots with sowing spaces 70x20, 70x10, 15x20 cm. It was shown that under such sowing patterns, the plants have produced up to 81.51 t/ha of biomass and about 57.9 t/ha of stalks. It is worth noting that the higher the sowing density was, the lower were the yields, except for the 15x20 variant, which provided 79.98 t/ha of biomass. On the other hand, the density of plants sowing, in this case (15x20), reached 333 thousand plants per hectare, while for 70x20, it was at least twice lower (143 thousands/ha) and for 70x10, four times lower (71 thousand/ha). Therefore, taking into account the data of plants morphology (height and stem diameter), it can be assumed that a high biomass yield of 15x20 spacing variant may result in a high number of plants grown in the area. At the same time, higher sowing density (667 thousands/ha) has led to lower productivity because of generally worse plant growing conditions.
Spacing Between Rows x Plants in a Row, cm×cm | Plant Height, cm | Stem Diameter, mm | Number of Internodes on the Stem | Number of Leaves | Leaf Length, cm |
---|---|---|---|---|---|
70 ×30 | 322.4±4.79b | 15.8±0.54a | 9.6±0.34a | 9.8±0.42ab | 84.8±1.99b |
70×20 | 386.0±16.18a | 16.0±0.87a | 9.8±0.66a | 10.8±0.69a | 92.8±2.18a |
70 ×10 | 331.8±8.77b | 16.0±0.83a | 9.8±0.66a | 10.8±0.71a | 92.8±2.19a |
45 ×30 | 291.5±11.21c | 14.8±1.15a | 9.75±0.92a | 9.5±0.93ab | 86.0±3.13b |
45× 20 | 394.0±7.64a | 15.4±1.50a | 10.2±0.53a | 10.2±0.53a | 90.0±2.17ab |
45 ×10 | 381.0±10.47a | 11.0±0.85b | 10.8±0.27a | 10.8±0.27a | 88.8±2.54ab |
15× 30 | 232.7±12.50d | 11.7±0.98b | 7.0±0.56b | 6.67±0.49c | 61.3±1.93c |
15 ×20 | 276.3±14.06c | 10.0±0.45b | 7.25±0.16b | 6.5±1.29c | 63.3±1.06c |
15 ×10 | 212.2±13.85e | 6.40±0.37c | 7.0±0.45b | 6.4±0.29c | 61.6±8.76c |
Mean | 314.2±64.6 | 13.01±3.36 | 9.02±1.51 | 9.05±1.96 | 80.16±13.62 |
Spacing Between Rows x Plants in Row, cm×cm | Number of Plants, Thousands/ha | The Yield of Above-Ground Mass, t/ha | The Yield of Sugar, t/ha |
Estimated Ethanol Yield, L/ha |
---|---|---|---|---|
70 ×30 | 48 | 49.76c | 4.87c | 2084.1c |
70×20 | 71 | 77.22a | 7.55a | 3234.2a |
70 ×10 | 143 | 81.51a | 7.97a | 3413.8a |
45 ×30 | 71 | 51.06c | 4.99c | 2138.5c |
45× 20 | 111 | 64.38b | 6.3b | 2696.4b |
45 ×10 | 222 | 59.94b | 5.86b | 2510.4b |
15× 30 | 222 | 50.65c | 4.95c | 2121.3c |
15 ×20 | 333 | 79.98a | 7.82a | 3349.8a |
15 ×10 | 667 | 61.32b | 5.99b | 2568.2b |
Mean | 210 | 63.98±12.58 | 6.26±1.23 | 2679.6±535.7 |
The sugar content did not significantly vary either in biomass or in juice in all tested plots. Therefore, estimated sugar yield depended mainly on general stalk productivity. In the most productive sowing variants (70x20, 70x10, 15x20), it varied in the range from 7.55 to 7.97 t/ha. Thus, the potential ethanol yields were 3234.2-3413.8 L/ha for these variants. In other cases, potential ethanol productivity was lower, while the mean rate for all row spacing experiments was 2679.6 L/ha. In short, the conventional sowing patterns 70-75 cm × 20-10 cm appeared to be more effective for sweet sorghum cultivation (bearing in mind the conditions of Northern-Central Ukraine) due to the possibility to generate higher biomass/stalks/sugars/ethanol yield per hectare.
Similar observations were reported by other researchers since the nutrition area (or sowing density) significantly affects the growth of all plants [22, 29, 31, 32, 46]. The optimal sowing density (the number of plants per hectare) was suggested at about 116 thousand [52], which corresponds to 70x10, 70x15 or 75x10 cm row spacing variants that are commonly used [36]. Otherwise, narrower rows and higher sowing density led to the formation of thinner stems, making plants more conductive to lodging [52]. This effect has also been observed in the current research. An alternative solution is to sow sweet sorghum in clumps (3-5 seeds per single hole) with 50 cm spacing between each one of them. Such an approach will result in thickening of stalks, better irrigation of growth area, reduced lodging and similar yields, compared to the conventional sowing practice [53].
3.3.3. Soil Supplementation
The following experiments focused on the identification of soil supplementation impact on sorghum growth efficiency. For these trials, cv. Botanichniy was used with 70x10 cm sowing pattern, which was found to be the most effective for cultivation. The influence of pre-sowing treatment of seeds with bactericidal preparations or application of fertilizers on sweet sorghum productivity was found to be significant (Table 8). The results showed that the biomass yield significantly increased, compared with the control plots. The highest biomass productivity was identified with phosphobacterin and N-P-K supplied plants, with 124.4 t/ha and 112 t/ha values, respectively. Combination of biopolycide and phosphobacterin pre-treatments showed lower biomass accumulation than phosphobacterin supplementation alone. Previously, it was reported that under appropriate climate conditions, sweet sorghum was able to generate biomass yields, sugar content, sugar yield and ethanol production similar to sugar cane; it can provide yields of more than 100 t/ha of biomass (total biomass weight, except seeds) on average. These characteristics of sweet sorghum make it more preferable in comparison with traditional sugar beet [1, 5]. Usually, yields of the total above-ground mass vary from 60 to 120 t/ha, while minimal seed yield is 900-1800 kg/ha depending on the genotype [15, 27, 30].
Supplementation Type | Total Biomass Yield, t/ha | Total Dry Matter Yield, t/ha | Sugar Content in Biomass, % | Estimated Ethanol Yield from Juice, L/ha |
---|---|---|---|---|
Control (None) | 64.4e | 25.8c | 12.8b | 2646.2c |
Biopolycide | 83.3d | 34.2b | 13.09ab | 3507.1b |
Phosphobacterin | 124.4a | 43.4a | 13.56ab | 5408.6a |
Biopolycide + phosphobacterin | 98.8c | 36.8b | 11.61c | 3651.5b |
N-P-K 16-16-16 | 112.0b | 44.3a | 14.12a | 5098.4a |
Mean | 96.58±22.25 | 36.9±7.16 | 13.04±1.03 | 4062.3±1158.5 |
Supplementation Type | Grain Yield, t/ha | Estimated Ethanol Yield from Grain, L/ha |
---|---|---|
Control (None) | 5.06c | 1599.4c |
Biopolycide | 6.51a | 2057.8a |
Phosphobacterin | 6.73a | 2127.3a |
Biopolycide + phosphobacterin | 5.79b | 1830.2b |
N-P-K 16-16-16 | 5.94b | 1877.6b |
Mean | 6.01±0.65 | 1898.5±207.5 |
Dry matter content did not vary significantly (mean value - 38.4%), contrary to dry matter yield, which depended highly on general biomass productivity. At the same time, Cséfalvay et al. [54] established that in stems of cultivar Sucrosorgho-506, the content of dry matter could reach only 24.54% w/w, while Mahmood et al. [55] showed that it could vary from 21 to 32%. Additionally, Wu-tai et al. [56] reported that fresh sorghum at the harvest time contained 30.3% w/w of dry matter.
Sugar content in biomass did not significantly differ from control (12.8%), biopolycide (13.09%) and phosphobacterin (13.56%). At the same time, combined pre-treatment (phosphobacterin + biopolycide) showed a significantly lower rate of sugar accumulation in biomass, i.e. 11.61%. Conventional N-P-K treatment significantly outweighed the control value and reached 14.12% of sugars in biomass. In this case, potential sugar yield reached 8.98 t/ha, while in one of the replications (of N-P-K supplementation experiments), the value of 9.5 t/ha was identified, which was the maximum sugar yield in this study. Also, another study of the sweet sorghum juice showed that the content of total carbohydrates was 11.84–20.34% w/v, depending on years [54]. At the same time, Silva Ferreira et al. found the total content of reduced sugars in biomass as 11.67–13.05% [57], while another study suggested that sugar content in juice varied within the range of 10.39–16.93 Brix% [32]. In our study (in supplementation experiments), sugar content in the juice of cv. Botanichniy was 18.3-23.3 Brix%. Mahmood et al. [55] reported similar maximum content of sugar in the juice of sorghum hybrids up to 24.3%. However, these values are highly dependent on genotype peculiarities. Another sample of S. bicolor cv. Wray had stem yields of 47.9–65.9 t/ha and sugar yield of 2.86–4.01 t/ha [58]. Investigation of Turkish sorghum cultivars established juice output of 8728–35143 L/ha and total sugar content of 0.61–6.38 t/ha depending on the location [12].
Finally, the potential ethanol yield has been estimated for each supplementation variant. Mean ethanol yield for all supplementation experiments (excluding control) was 4416.4 L/ha. The highest ethanol yields were identified within N-P-K supplied plots – 5098.4 L/ha and within phosphobacterin pre-treated ones – 5408.6 L/ha. Additional ethanol could be produced from sorghum grain, whose yield has also been estimated under the same soil supplementation experiments (Table 9), using our proposed equation (7). It is interesting to note that in terms of grain yields, bacterially (solely) pre-treated plants showed better performance (6.51-6.73 t/ha) than N-P-K supplied (5.94 t/ha). Similar to biomass productivity, plants pre-treated with a combination of bacterial inoculants showed lower grain yield than separate supplementation of biopolycide or phosphobacterin. Respectively, an additional ethanol output up to 2127.3 L/ha can be produced by converting this grain (phosphobacterin supplementation). Mean ethanol yield for all supplementation experiments (excluding control) was 1973.2 L/ha.
According to the other reported findings, the optimal N-P-K supplementation for sweet sorghum varied from 90 to 120 kg/ha, which might in some cases double the yield [59]. In addition, the authors of these findings revealed that under their specific climate conditions (Semi-Arid Tropical Zone in India), a higher supplementation rate (up to 150 kg/ha) did not cause a significant effect on the yields. Other researchers suggested that 165 kg/ha supplementation was required for optimal sorghum productivity [36]. According to other research findings, under the conditions of Northern Great Plains (USA), nitrogen supplementation is more crucial for sorghum cultivation. It is required at the rate of at least 100 kg/ha but can be applied at 180 kg/ha for an even higher increase in biomass productivity [60]. The same effect was also observed by other researchers [61], suggesting that nitrogen fertilization could be extremely important to increase the sorghum yields.
In the present study, we have shown that NPK supplementation at the rate of 135 kg/ha provided the highest yields. In the case of cv. Botanichniy, the effect of pre-treatment with P. polymyxa was similar to the effect of 60 kg/ha N-P-K supplementation (Tables 3 and 8). Interestingly, a combination of P. polymyxa and B. megaterium pre-treatments showed higher biomass productivity, compared to biopolycide sole application, although the increase was lower in case of treatment with phosphobacterin. The reason for such effect remains to be investigated since its mechanism is unknown, though it may be associated with a competitive inhibition between these bacterial species.
3.3.4. Biomass By-products and their Utilization
An additional study was conducted to determine the energetic value of biomass before juice pressing (fresh stalks) and afterward (bagasse) (Table 10). In most of the cases (except the AMBR-2), an increase in calorific value of kg of biomass after juice pressing was observed, which can be explained by a high loss of moisture. The energetic value of biomass was significantly different among most of the genotypes. The mean calorific value of fresh biomass was 3647.8 kcal/kg, while this value for bagasse was 3854.02. The mean rate of caloric value change was +206.2 δkcal/kg (±311.6), while this value excluding AMBR-2 was +312.4 δkcal/kg (±191.6). The sugar content also varied amongst different cultivars and lines. Thus, cv. Botanichniy, Medove, Yantar possessed 20.58-21.2% of sugar in juice, which was significantly higher than the values identified for AMBR-5 and cv. Bilotsukrova – 18.31-18.78%. The lowest sugar content of only 14.05% was found in the juice of AMBR-2. No significant correlations (Pearson’s) were found either between energy value and sugar content or among δkcal/kg and juice content. At the same time, two-way ANOVA test conducted has shown that the influence of genotype factor (F=2.72, p<0.05), juice pressing factor (F=10.84, p<0.01) and interaction between them (F=4.13, p=0.008) were significant. These findings indicate that change of caloric value, as well as the resulting energy value of bagasse, may highly depend on the genotype peculiarities and should be analysed individually in each separate case.
The differences in calorific value can presumably be caused by alternations in monosaccharide composition of bagasse. For example, it was found that the content of xylose can be significantly different within the sweet sorghum varieties [45]. A large amount of biomass by-products are left after the use of sweet sorghum stalks for juice pressing and further ethanol production. Bagasse can be utilized in a number of possible ways: biogas production [62], soil fertilizers [63] or production of pellets and briquettes for burning [64], etc. Additionally, both sorghum bagasse and molasses can be potentially used for biobutanol production [65].
On the other hand, another important and promising direction is bioethanol production out of such cellulosic feedstock, which can provide an additional amount of ethanol, comparable with yields of juice-derived alcohol [45]. The study of sweet sorghum samples in Poland reported that raw biomass contained sugars in the amount of 72.26 g, cellulose - 279.52 g, lignins - 28.16 g and crude ash 48.86 g per 1 kg of dry weight [55]. Therefore, both sorghum biomass or bagasse can be used as efficient feedstocks for bioethanol production. Otherwise, such approaches require rather different and more sophisticated technological procedures than conventional juice/grain ethanol production.
3.4. Evaluation of Potential (Bio)Ethanol Productivity
Different methods of ethanol yield estimation can usually give only a general view of the potential productivity of technical alcohol production. The influence of the method of calculation factors (Smith et al. [37], equation (5)) on the final results is presented in Fig. (4a). The highest recorded productivity rate in this study was 9.5 t/ha, which was chosen as the reference value of sugar yield. This particular equation is based on the sugar productivity value, to which a conversion index is applied (0.665 L ethanol per 1 kg of sugars).
Also, the production efficiency coefficient was assumed to be 0.8 (80% of production efficiency), which takes into account losses during the real production process. Respectively, having applied the production efficiency rate (0.8) to the conversion rate (0.665 L/kg), the resulting conversion rate was 0.532 (L ethanol per 1 kg of sugars). Such an approach was used in equation (6) proposed by Somani et al. [38]. However, the conversion rate of 0.665 L/kg is a mean value of corresponding rates for sucrose (0.682 L/kg) and hexose (0.648 L/kg). The influence of different conversion rates on the final estimation is shown in Fig. (4a). Thus, the mean conversion rate suggests that the potential ethanol yield can be 5054 L/ha. Likewise, if the hexose coefficient is applied, this value can be 4924.8 L/ha, and in the case of sucrose, the value can become 5183.2 L/ha. Although such divergences may not seem to be significant in calculations, they could have serious implications in the case of estimating the efficiency of real large-scale production. It can therefore be concluded that for the sake of more accurate estimation, sugars composition for particular sweet sorghum genotypes should be investigated. This would enable the ultimate correction of the coefficients. Besides that, the equation (5) proposed by Smith et al. [37] was tested against real fermentation experiments (under laboratory conditions with 250 mL of juice) and proved to be accurate enough for estimations in the present form [36]. Otherwise, larger-scale production may require an even higher level of accuracy in calculations.
Genotype | Energy Value Before Juice Pressing, kcal/kg | Energy Value After Juice Pressing, kcal/kg | Sugars Content in Juice, % w/w |
---|---|---|---|
AMBR-2 | 3675.7bc | 3350.73c | 14.05c |
AMBR-5 | 3552.7c | 4075.62a | 18.78b |
Bilotsukrova | 3699.86b | 3949.53ab | 18.31b |
Botanichniy | 3572.44bc | 3865.88b | 21.2a |
Medove | 3541.23c | 4001.22a | 22.45a |
Yantar | 3845.11a | 3881.14b | 20.58a |
Mean | 3647.84±117.05 | 3854.02±258.49 | 19.23±2.96 |
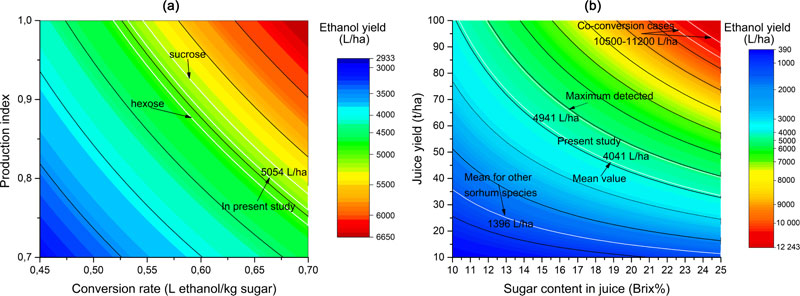
In Fig. (4b), the impact of sorghum productivity parameters on the estimation of final ethanol output is shown. This figure provides a graphical interpretation of the equation (6) proposed by Somani et al. [38]. This calculation method takes into account the fact that not all soluble substances in the juice are sugars, identifying their content as Brix%-3. However, usage of the constant value (of -3) for normalization of sugars content level leads to a significant distortion of the estimated ethanol yield. Thus, the constant value subtracted from low Brix% makes a more significant impact on the ultimate calculation result than the same operation done for high Brix% values (approx. ≥20%). Similar peculiarities of this estimation method were also discussed by Bunphan et al. [36]. This effect will be the most tangible in case of very high juice yields (blue zone on Fig. 4b). If extremely high ethanol yield values are considered, the data obtained with this equation will show linear dependence. Indeed, such high values (over 5000-6000 L/ha of ethanol) could take place extremely rarely or almost impossible in practice. The maximal actual ethanol yield in a soil supplementation experiment was 4941 L/ha, while the calculated mean rate was 4041 L/ha (excluding control- without soil supplementation). At the same time, the mean value for other different S. bicolor subspecies (or other species) was only 1396 L/ha caused by a concurrent combination of low juice yield and low sugars content in it. However, this mean figure excludes the value of S. durra x saccharatum hybrid, which demonstrates the average productivity compared to its parental species (subspecies).
Similar to the previous equation (5), this calculation method (6) was also tested against real ethanol production experiments and has shown a desirable accuracy [36]. In general, both equations (5 and 6) provided almost similar values of ethanol yield, except in the cases where sugar content was high (≥18%) as well as juice yield (over 30 t/ha). The difference was only 1.4-3.6% when the equation (6) provided higher values. In the cases when the actual input productivity data was higher than indicated, this difference rose to 4.8-5.7% or more. When the juice yield was below 20 t/ha and, at the same time, the sugar content was lower than 15%, equation (6) gave lower ethanol yield values than equation (5). The difference in this case was 3-6.1%. However, both equations (5) and (6) provided significantly lower values than equation (4), proposed by Lipinsky [35], which was based on stalks yield. The equation by Lipinsky [35] assumed higher production efficiency (85%) and used sugars % in stalks for calculation. It also used the same conversion rate of 0.665 L/kg, but did not take into account the potential losses during juice pressing since that formula dealt with stalk productivity parameters instead of juice or sugar yields. However, it also provided linear dependence of data derived from it, similarly to equation (5), thus dealing equally well with high or low yield values.
The equation (4), based on the stalks yield, was not tested in ethanol production experiments. Besides, the calculations by that method provided much higher ethanol yields – up to 5000-5500 L/ha [34] or even higher – above 7000 L/ha of ethanol form juice [66], what seemed to be, probably, rather overoptimistic, although theoretically possible. In the present study, the equation by Lipinsky [35] also provided significantly higher values of ethanol yield, compared to the equations (5) and (6). At the same time, the practical verification of equations’ accuracy, mentioned previously [36], showed significantly lower values of ethanol yields, calculated via equation (6) – only up to 3000 L/ha. However, these studies present the results of sorghum productivity trials under diverse climatic conditions (precipitation, mean temperature, etc.) that significantly impacted upon the resulting data. Moreover, humidity, precipitation and temperature fluctuations can also significantly impact on the sweet sorghum productivity since this crop is well resistant to semi-low watering conditions [47].
Thus, in the present study, the ethanol yield was calculated as a mean of the three mentioned equations (4-6). The value of 4416.4 L/ha was established as a final reference value of ethanol yield from sweet sorghum juice that is possible to obtain under the climate conditions of Northern-Central Ukraine (Forest-Steppe zone, temperate climate zone). This value is the mean of ethanol yield from the soil supplementation experiments (Table 8), except for the control.
Additionally to all the above-mentioned peculiarities of ethanol production rates estimation from sorghum juice, the great perspective is held by co-conversion of the whole plant biomass into ethanol and bioethanol. Potential yields may rise up to 10500 L/ha [45] or 11200 L/ha [67]. Fig. (4b) indicates a red zone of ethanol yields that can be achieved if sorghum juice, grains and bagasse are converted into ethanol. Such an approach could allow obtaining a huge amount of ethyl alcohol, compared with a conventional sweet sorghum juice fermentation, since the latter will require a rapid increase in plant productivity that cannot realistically be achieved at present.
Our estimation of potential co-conversion of different sorghum raw materials into ethanol is shown in Fig. (5). The ethanol yield from sorghum grain was determined at the level of 1973.2 L/ha (mean from the soil supplementation experiments, excluding control, Table 9), which was estimated by equation (7). The resulting yield of ethyl alcohol from both grain and juice feedstock was 6389.6 L/ha, which is very close to the values determined by Castro et al. for cv. UF15 [45].
To evaluate the potential amount of bioethanol that can be produced from sweet sorghum bagasse, another calculation method has been applied (equation 8), which is based on an average rate of conversion of dry SSB into bioethanol, involving various pre-treatment procedures. SSB amount was estimated on the basis of the data resulting from soil supplementation experiments (excluding control). Estimation of bioethanol yield suggested that it was possible to obtain additional 5033.5 L/ha of EthOH from SSB. However, the SSB/bioethanol conversion rates were very different for technologies involving various biomass pre-treatment methods. Thus, bioethanol production with acidic pre-treatment [44] may allow to produce only 4467.1 L/ha, while SO2 steam explosion pre-treatment [42, 43] will result in even lower yields – 3957.3 L/ha. A novel approach that involves steam explosion impregnated with H3PO4 pre-treatment and following transgenic E. coli fermentation [45] could provide significantly higher bioethanol yields – up to 6676.4 L/ha. On the other hand, not all the technologies mentioned can be easily applied for industrial-scale production with the same efficiency.
According to our estimation, the total (bio)ethanol output that can be produced from the combination of juice+grain+SSB is 11423 L/ha. The calculated value is not significantly different from other mentioned estimations suggesting 10500-11200 L/ha of ethanol yield, resulting from such co-conversion approach [45, 67]. Moreover, another recent research revealed that it was possible to produce up to 10344 L/ha of bioethanol from sweet sorghum stems only if juice was not preliminary pressed [68]. Such evaluation is also consistent with our elaborations and other research findings since adding grain ethanol to the value estimated by Byrt et al. [68] could increase the calculated value by only 1000-2000 L/ha. Therefore, the resulting total amount of estimated (bio)ethanol yield will be close to either our estimation or the ones suggested by Barcelos et al. [67] and Castro et al. [45].
Apart from this, obtaining ethanol from a combination of juice+grain seems to be more realistic for industrial-scale production, since bioethanol production may have hardly-predictable economic efficiency. However, the productivity of SSB conversion into bioethanol can be further improved by using xylose-fermenting organisms, such as Trametes versicolor [69, 70] or Hansenula polymorpha [71]. Another approach, which could allow increasing the efficiency of ethanol production from sweet sorghum is associated with the genetic engineering of this species. As an example, manipulations with SWEET genes could be considered to enhance sucrose accumulation in stalks [72]. Mutant sorghum lines are also regarded as promising biofuel feedstocks with increased sugars content (ARS14 mutants) [73] or reduced lignin content (bmr mutant hybrids) [74].
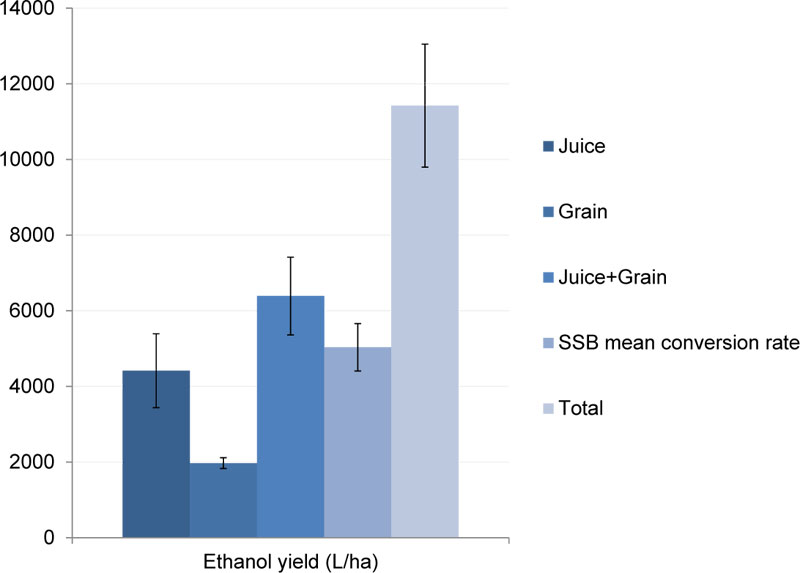
Furthermore, recent advances in molecular genetics allow obtaining lipid-producing (in biomass) sorghum [75]. Co-conversion approaches for biofuel obtained from lipid-producing sorghum have already been proposed [76]. Additionally, a technology involving lipids transesterification with bioethanol for biodiesel production has been investigated [77]. As reported earlier [24], dry, pre-treated sorghum bagasse can be co-fermented with juice and with other biomass feedstocks such as finger millet (Eleusine coracana) [78]. Alternatively, sweet sorghum bioethanol can serve as an energy source for the production of conventional juice and grain ethanol. Finally, conventional breeding also offers promising opportunities. For instance, wild Sorghum species can serve as a donor of genes (allele variants) associated with high-stress tolerance [79].
Also, further improvement of sweet sorghum through its breeding can be extended using the existing broad gene pool, which was evaluated under the present study, as well as other sorghum germplasm, genetic diversity of which has already been characterized [49].
CONCLUSION
Sweet sorghum has considerable potential in Ukraine as a new sugar-producing energy crop. This has been revealed by the outcomes of prolonged research in the country. Sweet sorghum germplasm collection has been created (41 accessions), including introduced and acclimated genotypes and newly bred lines and varieties. The biological performance of sorghum under climate conditions of Northern and Central Ukraine and plant morphology have been analyzed. Additionally, optimal cultivation conditions (70x10 cm row spacing, fertilization rate at 135 kg/ha of NPK 16-16-16) for sweet sorghum have been identified as well as productivity of different breeding lines and cv. Botanichniy has been evaluated. Based on the obtained data, the potential (bio)ethanol yield for different sugars feedstocks (juice, grain bagasse) has been estimated. The obtained results suggest that it is possible to produce 4041 L/ha from juice and up to 6389.6 L/ha from a combination of juice- and grain-derived ethanol yields. Additionally, the utilization of bagasse may increase the overall (bio)ethanol yields up to 11423 L/ha. The estimated ethanol productivity is comparable with other similar investigations. It signifies the high performance of created sweet sorghum under the conditions of Northern and Central Ukraine and provides additional evidence of the accuracy of the conducted estimations.
ETHICS APPROVAL AND CONSENT TO PARTICIPATE
Not applicable.
HUMAN AND ANIMAL RIGHTS
No animals/humans were used for studies that are base of this research.
CONSENT FOR PUBLICATION
Not applicable.
AVAILABILITY OF DATA AND MATERIALS
Not applicable.
FUNDING
This work was supported by the National Academy of Sciences of Ukraine (Projects No. 374-NK, State registration No. 0114U002193 and Projects No. 8-17, State registration No. 0113U002925).
CONFLICT OF INTEREST
The authors declare no conflict of interest, financial or otherwise.
ACKNOWLEDGEMENTS
We acknowledge the suggestions by Dr. V. Kyrylenko (Institute of Food Biotechnology and Genomics, National Academy of Sciences of Ukraine, Kyiv) for improving the manuscript language.