All published articles of this journal are available on ScienceDirect.
Efficiency of Switchgrass (Panicum virgatum L.) Cultivation in the Ukrainian Forest-Steppe Zone and Development of Its New Lines
Abstract
Background:
Switchgrass (Panicum virgatum L.) is a promising C4-photosynthetising perennial grass and an important energy crop. It is resistant to drought, cold and winter frost, endures flooding and shows high performance at minimal energy consumption for cultivation.
Methods:
The purpose of the work was to develop and introduce high-yielding genotypes of switchgrass and develop effective cultivation technologies for the Forest-Steppe zone of Ukraine. The objective of the study was to evaluate the introduction potential of switchgrass, to establish biologically productive parameters and energy values of different genotypes and the effectiveness of cultivation techniques in the specific geo-climatic zone of Ukraine. To achieve the objectives, a wide range of introductory, biological-morphological, breeding-biotechnological, and agronomic methods have been used.
Results:
The gene pool of P. virgatum was collected (33 accessions), ten of which have been investigated, including both introduced genotypes and newly developed breeding lines. The bio-morphological, ecological features, the biochemical composition of plants have been evaluated. The productivity of the above-ground biomass and seeds, as well as the energy value of plants, has been evaluated. High-performance forms with specified yield parameters, biomass sugars, total energy and biofuel output per unit area have been determined.
Conclusion:
A new variety of P. virgatum (cv. Zoriane) has been introduced for cultivation. Among the accessions of newly developed genotypes, the most productive lines have been identified. New efficient technologies have been developed to increase the yield potential of switchgrass as a source of raw materials for biofuel production.
1. INTRODUCTION
Renewables are widely believed to make a significant contribution to the world's energy balance in the foreseeable future. In this context, biofuels are becoming an energy resource of growing importance. Within the plant family Poaceae, there are some high-yield and cost-effective sources of biofuels [1], similar to other promising perennial energy crops of the genus Panicum L [2-4]. Among those, switchgrass (Panicum virgatum L.) deserves special attention. In the wild flora, it occurs from the latitude of 55° to the north in southern Canada, the United States and Mexico, and is one of the dominant species in the central North American prairies. The high productivity and perennial nature of switchgrass enable the plantations to be retained for decades. Moreover, unlike corn or millet, switchgrass can grow on nutrient-deficient soils [5-9].
Switchgrass refers to the model energy crop with high aboveground productivity that can be considered a real source of renewable fuels and electricity [10]. Switchgrass is identified as an important source of fuel production from cellulosic feedstock. High yields and adaptability to some unfavorable environmental conditions determine, under the right management practice, its beneficial economic, environmental and social potential of its cultivation [11, 12], given the growing interest for new biofuel crops [13-17].
Unlike traditional crops, switchgrass does not require annual renewal and use of pesticides and fertilizers [18, 19]. It is a thermophilic plant reproduced by both seeds and rhizomes. Switchgrass has an efficient root system, capable of penetrating up to three meters deep, and grows as a dense shrub of 90 to 250 cm, depending on the genotypes. This plant can grow in different soils – from light sandy to heavy clay soil and from dry to moderately moist. Unaffected by soil acidity, switchgrass can grow at a рН range from 4.6–7.6. Thus, switchgrass can be cultivated on lands not suitable for other crops. This crop is highly resistant to diseases and pests.
Over the recent years, significant attention has been paid to the biochemical composition of plants, which reflect the peculiarities of the accumulation of certain compounds and determine the important components and characteristics of their raw materials: calories, nutrient content, ash, and macronutrients, as well as antioxidants presence. All these parameters depend on the genotype and may vary from cultivar to cultivar [20-22]. Switchgrass possesses a valuable chemical composition of aboveground mass, which is typical for biofuel biomass: about 50% carbon, 43% oxygen, and 6% hydrogen. Plants contain 4–6% ash due to a high proportion of leaf mass. A relatively low content of potassium and sodium, combined with a high content of calcium and magnesium in biomass, determines the high combustion temperature and a low likelihood of slagging when burned in boilers [23]. The main components of switchgrass biomass are cellulose (35%), hemicellulose (29%) and lignin (26%) [24, 25]. A single harvest per year provides a sufficient amount of cellulose and lignin [26]. Switchgrass can be used as a cellulosic feedstock for biofuel production, as soil cover for its conservation and erosion prevention, as forage for grazing, as a technical culture for mulching, and as a substrate for mushroom cultivation [24, 27]. In addition, co-cultivation of switchgrass with the ectomycorrhizal fungus under drought condition resulted, in comparison with control plants, in significantly higher biomass productivity and increased macronutrient content [28].
The chlorophyll content is an important feature of this plant to tolerate different temperature conditions [29, 30]. Switchgrass with a C4 photosynthesis scheme is a high-yielding bioenergy crop and is a valuable source of sugars for bioethanol production [23, 31-33]. It was also reported that switchgrass cultivation required a lower energy supply compared to other cereals. Only 0.97-1.34 GJ were required for the production of 1 t of switchgrass straw material vs. 1.99-2.66 GJ needed for the production of 1 t of the biomass of other grain crops [34]. Another study has shown that switchgrass cultivation requires 0.8 GJ energy input from fossil fuels per 1 t of the dry weight, which is significantly lower than 2.9 GJ per 1 t of dry weight needed for corn biomass growing [35]. Switchgrass’ biomass contains about 18.8 GJ of energy per 1 t of absolute dry weight, which provides almost 20:1 return of energy, compared to inputs, spent on its production. These data indicate an extremely efficient correlation between energy generated with a yield of 1 ha and low energy costs for its production. The sustainability and eco-friendliness of switchgrass-based ethanol production could be further increased with the use of alternative variety or by optimizing soil conditions [16].
In Ukraine, previous switchgrass studies covered issues of agricultural technology, seed and biomass yield, reproduction, the biochemical composition of its raw materials, development of high-performance forms and varieties and other aspects [2, 36-38]. As a result of the many years of research, for the first time, agrotechnology of switchgrass cultivation for biofuel production has been adopted for the area of the Forest-Steppe of Ukraine. The patterns of plant growth and development under the influence of abiotic factors were established and enabled to optimize technological measures to improve the biomass productivity of this species [2, 38, 39]. The scientific and methodological background, developed in Ukraine, allows the computer-aided modeling, based on the correlation between biological parameters, the actual yield of dry biomass and optimal growing conditions to be applied for the improvement of energy crop cultivation technologies, including switchgrass [40, 41].
An important role in research and development of introduction, breeding and cultivation technologies of energy crops of the Panicum genus has been played by M.M. Gryshko National Botanical Garden (NBG) of the National Academy of Sciences of Ukraine (Kyiv). The richest gene pool of Panicum accessions has been created at the NBG after many years of research. The high-yield switchgrass forms and varieties, bred at the NBG, are well suited for a sustainable biomass feedstock supply required for the production of second-generation bioethanol [38, 42].
The species in Panicum are tolerant of drought as well as cold, frost and flood. These plants have strong adaptability potential and can grow effectively in various temperate climatic conditions. For this reason, they are promising energy crops for the Ukrainian climate conditions. However, despite decades of research, comprehensive studies on the biological, ecological, biochemical features of these plants to develop high-yielding varieties adapted to local conditions still remain to be conducted.
Therefore, the aim of this work was to evaluate the introduction potential of switchgrass plants to establish biologically productive parameters and energy values of different genotypes and the effectiveness of cultivation techniques in a specific geo-climatic zone of Ukraine.
Additionally, the results of the assessment of switchgrass genotypes productivity were summarized, as well as the most promising genotypes, amenable for the development of new varieties, were identified. The results of the study provide insight into biomass production of switchgrass under the climatic conditions of Ukraine and shed light on the possible approaches for more efficient utilization of this crop.
2. MATERIALS AND METHODS
2.1. Plant Material
Representatives of the Panicum genus were first introduced under the conditions of Northern Ukraine at the Department of Cultural Flora at the NBG in 1995. Investigation of the switchgrass potential as a biofuel plant under laboratory and field conditions has been carried out since 2004. Switchgrass introduction studies and breeding experiments on the development of new forms and varieties for bioenergy use were first launched in 2007 and are still ongoing. During this period, a valuable switchgrass gene pool has been created (Fig. 1). The selected forms and varieties of switchgrass are characterized by early maturing, drought resistance, high yield of phytomass and seeds. As a result of the successful breeding work, the variety of Zoriane has been marked with the Ukrainian state certificate (Fig. 2) [43].
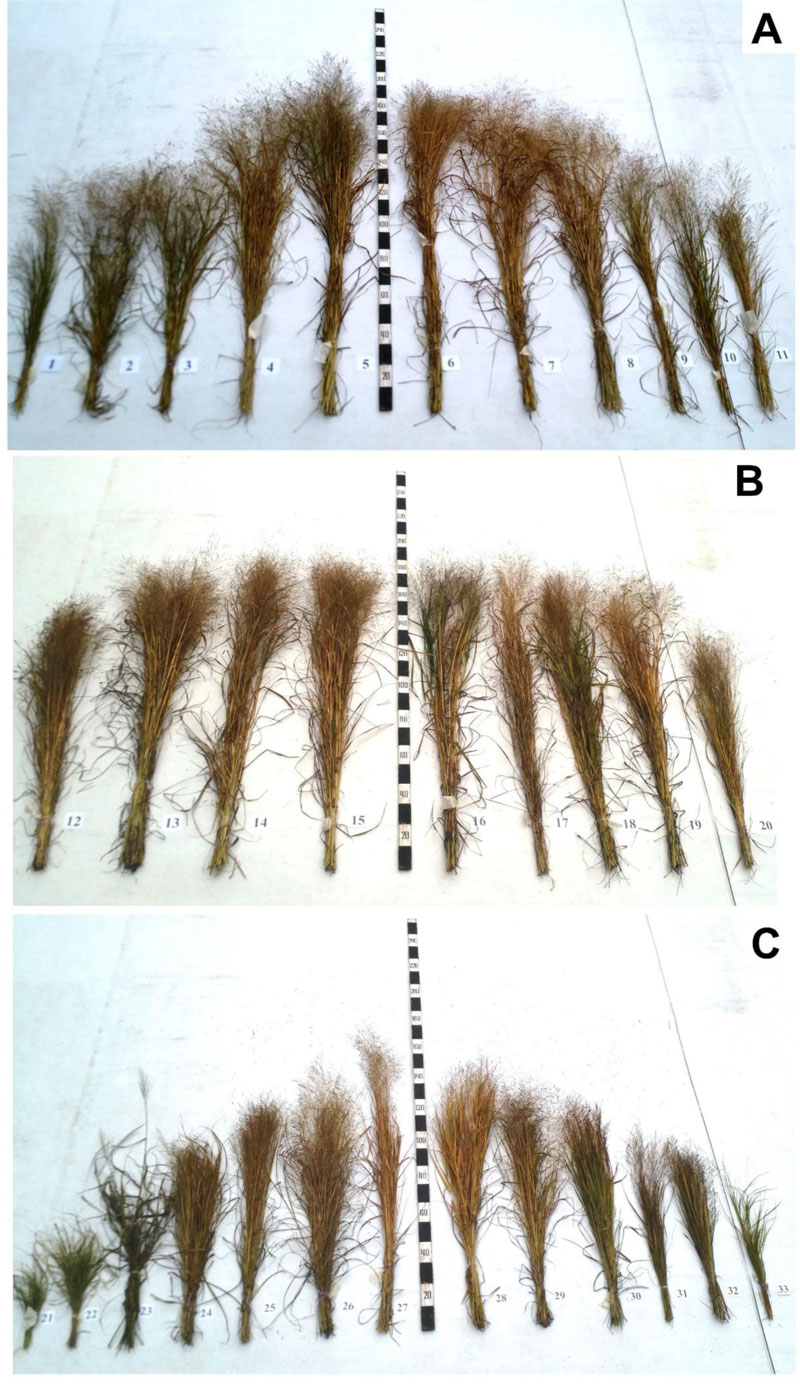
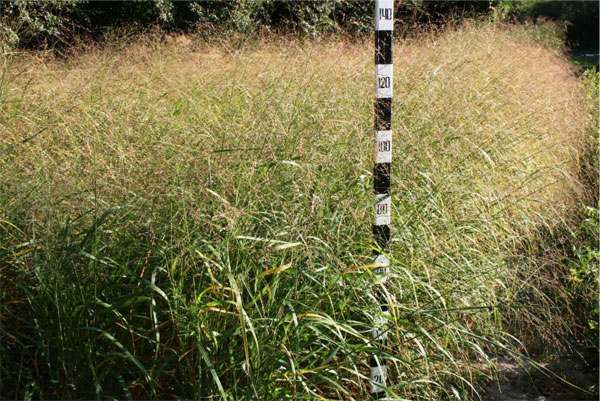
2.2. Field Tests
The initial small ground-plots research was conducted at the NBG. Field trials were conducted at the Agricultural Experimental Production Institute of Plant Physiology and Genetics of the National Academy of Sciences of Ukraine “Hlevakha”. Production studies were carried out in the Kyiv region (50°16′06″N 30°19′15″E). The experiments were carried out according to the existing techniques for State variety network and research institutions lasting three to six years in four repetitions [44]. The sizes of the plots were 60-100 m2, area of growth and accounting of plants – 30-60 m2. Switchgrass seeds were sown on plots in rows (equally spaced) in a semi-randomized way to keep the same sowing rate within different genotypes. The number of repetitions was four for each genotype.
The climate of the area under study (Kyiv) is temperate continental. The average monthly temperature in January is -5.5°C, and +19.2°C in July. The absolute minimum was -32.2°C in February 1929, and the absolute maximum was +39.9°C in August 1898. The average annual rainfall is 649 mm, the maximum rainfall is in July (88 mm), and the minimum is in October (35 mm). Snow cover is formed in Kyiv in winter, the average height of the roof in February reaches 20 cm, and the maximum was 44 cm [45]. During the study period, the average monthly air temperature was higher than normal in almost all months (Fig. 3) [46].
According to long-term data, the average monthly rainfall during the growing season in the study area is 504 mm (Fig. 4). Measurements have shown that rainfall in 2014 was below normal, exceeding normal in May (91 mm).
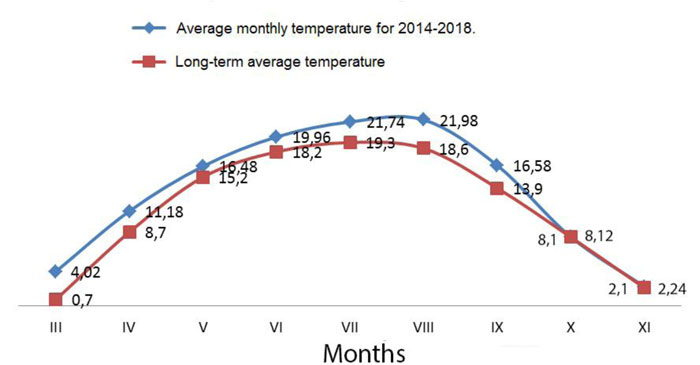
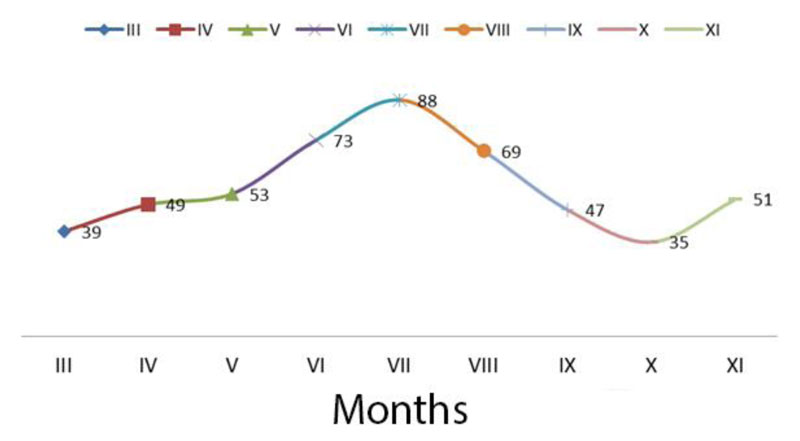
In 2015, during the growing season, the rainfall was below the norm except for May, especially in June and August. The arid period was from June to August, when the least amount of rain fell. In 2016, the precipitation was the highest in May, exceeding the norm by almost 270%. During June-September, on the contrary, there was a significant moisture deficit. The rainfall during this period was below the norm by 191-940 mm. Regarding the precipitation during the growing season, 2017 (except for October) was the driest. The lowest level of water delivered to the plants was observed in March, June, and July. During the 2018 vegetative period, rainfall exceeded normal during June and September. Due to the high adaptive potential and stability of the created forms and varieties of switchgrass, adverse weather and climatic conditions did not significantly affect the growth, development and production process.
For the purpose of this study, new forms (9) of switchgrass as well as newly developed variety (1) for Ukraine (from a total of 33 existing genotypes in the collection) were used [47]. In the current research, the following breeding lines and varieties of P. virgatum were examined: lines SL-1, SL-2, DN, PP, RB, RL, RR, VP, PL and сultivar Zoriane. Biometric measurements were performed [44]. The plant height and morphological description of their organs were evaluated by measurements and observations. Common morphological terminology was used to describe the morphological features of the structure of representatives of the studied plants [48]. All measurements were done in at least three repetitions (n=3).
2.3. Biochemical Analysis of Biomass
For biochemical analysis, samples were taken in the flowering, fruiting, and seed-maturing phases according to the conventional methods [49]. The determination of absolutely dry matter was done by drying to constant weight at 105 °С, followed by identification of weight reduction of samples (due to moisture evaporation) and calculating of dry weight content (% w/w). The total content of sugars was investigated by Bertrand’s method [50] in water extracts. The level of total ash was determined using the method of combustion in muffle-oven (SNOL 7.2-1100, Termolab, Ukraine) at 300-700 °С. The detection of energetic value was conducted on calorimeter IKA-200. Estimation of potential bioethanol production was done according to our previous study on P. virgatum [2]. All experiments were done in at least three repetitions (n=3).
2.4. Statistical Analysis
All statistical processing of the obtained data was conducted using OriginPro 9.1 software. Deviations of all means were calculated as a standard deviation (SD). To identify the significance of differences in different morphological and productivity parameters between the studied genotypes, one-way ANOVA was used, which included the calculation of Fisher’s least significant differences (LSDs). The LSDs were used to identify homogeneous groups for values of particular morphological or productivity parameters (at p<0.05 significance level). In some cases, SD of means is not denoted in tables (where it was considered unnecessary), since one-way ANOVA includes a comparison of the data using mean values and SD.
Two-way ANOVA was conducted to identify the significance of the influence of factor pairs (genotype-growth stage and genotype-plant organ) on a particular parameter. For verification of differences significance, LSDs were also calculated (at p<0.05 significance level). In all cases for which two-way ANOVA was conducted, homogenous groups for data were identified via one-way ANOVA, regardless of the second factor (growth stage or plant organ). This was done to simplify the visual comparison of particular values.
3. RESULTS AND DISCUSSION
It has been established that under the conditions of plant introduction under the described local climate, all forms of switchgrass undergo a complete cycle of development from seed to seed, starting from the first year of life (Fig. 5).
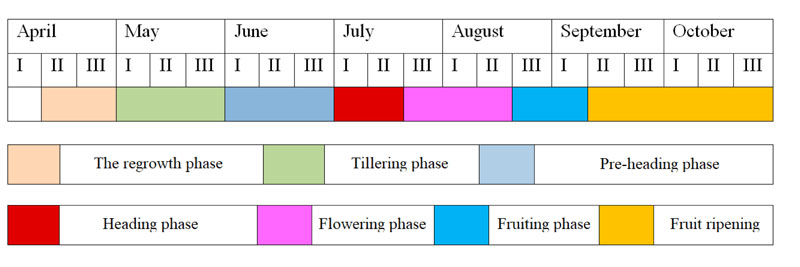
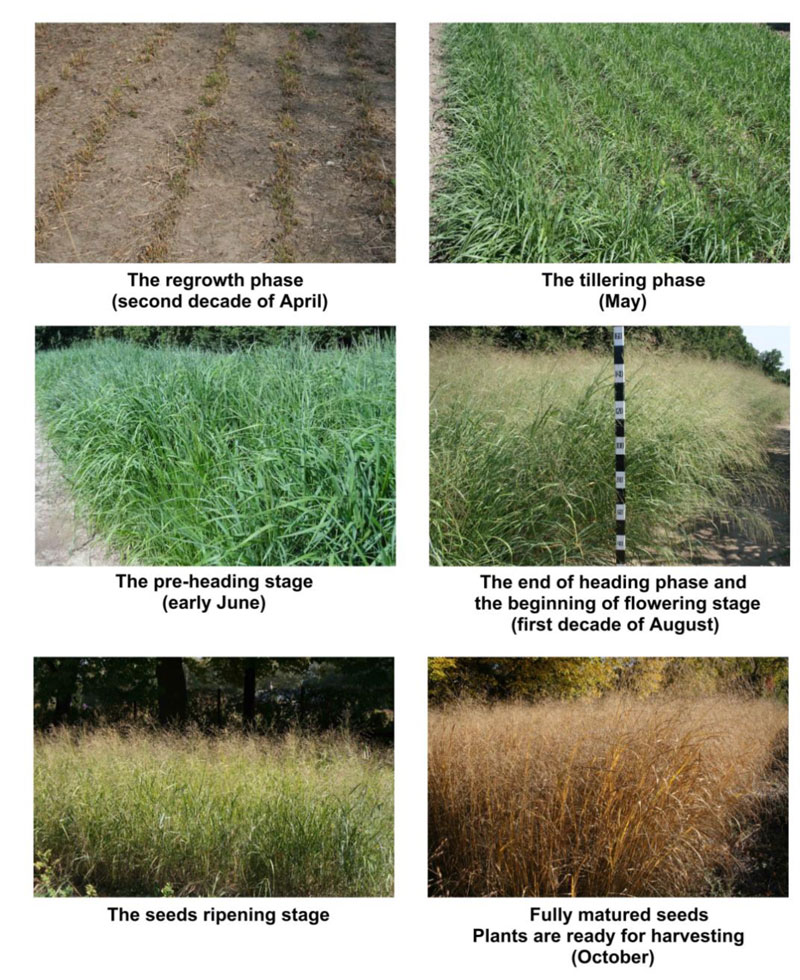
At the end of the growing season, the aboveground mass of switchgrass dies, however, regardless of genotype specifics. This plant was characterized by high resistance to winter conditions under the environment of the study. After wintering for the second year, in early spring, the intensive regrowth of plants begins (first ten-day period of April) and their further development occurs at different rates and ends in the autumn period with the formation of viable seeds (Fig. 6).
In the second ten-day period of April, the plants reach a height of 7-10 cm. In early June, the plants developed tillers and were 40-50 cm tall. By the first ten-day period of July, switchgrass plants grow intensively and reach the pre-heading phase. At this time, they were 90-120 cm tall. The intensive growth of plants lasts until the first week of August, when they have fully developed flowers. At this time, plants are 150 to 180 cm tall, while individual forms can be significantly higher - 210-220 cm. By the period of the beginning of the seed-ripening phase, the biomass growth rates of the plants are slowed down. In some forms, this phase occurred at different rates. Seeds of early-maturing switchgrass forms were fully ripened by the second ten-day period of September. Medium-mature forms grew until the third ten-day period of September. The development of late-maturing forms ends by the second or third ten-day period of October, while some forms are able to vegetate until the beginning of frosts (until the second-third ten-day period of November). It is interesting to note that plants of different forms of switchgrass are becoming very parti-colored by autumn (from golden-yellow to purple-brown).
During the first year of growth, switchgrass plants produced shoots very intensively. The number of basic productive stems on the plant in the period of generative development varied from 3-4 to 15 depending on the genotypes and growing conditions. During the second and subsequent years of growth, the number of productive shoots significantly increased from 12-14 to 30-35. The studied switchgrass accessions possessed different types of bush structure, in some cases, bushes were distinguished as erect, semi-spreading or spreading. The number of nodes on the stem varied from 3 to 7, while in some forms, their number could increase up to 10. The diameter of the stem base was approximately 4-6 mm.
The most of switchgrass genotypes are characterized by the absence of generative shoots branching. Only individual plants are able to form up to 4-6 lateral shoots on the main stem. The leaf lamina reaches 50-60 cm in length at an average width of 11-14 mm. The form of a panicle of different switchgrass genotypes can be classified as spreading, lump, oval, pyramidal or compressed (Fig. 7). The length of the panicle of switchgrass in different genotypes reaches 30-40 cm in length, 20-30 cm in width. Depending on the density, the panicles could be differentiated as low dense, medium dense and dense.
The analysis of studies of the biological and morphological features of switchgrass in Ukraine shows that the data obtained by us is unique for the conditions of our region [37, 39, 40].
During the first and the second years of growth, the formation of plants and a gradual increase in all their growth parameters were observed. Plants reached the highest morphometric parameters in the third year. During the period of technical ripening, a significant difference in morphometric parameters was established between the studied switchgrass accessions. According to the basic growth characteristics of plants, such forms of switchgrass were noted as f. VP and f. PP (Table 1).
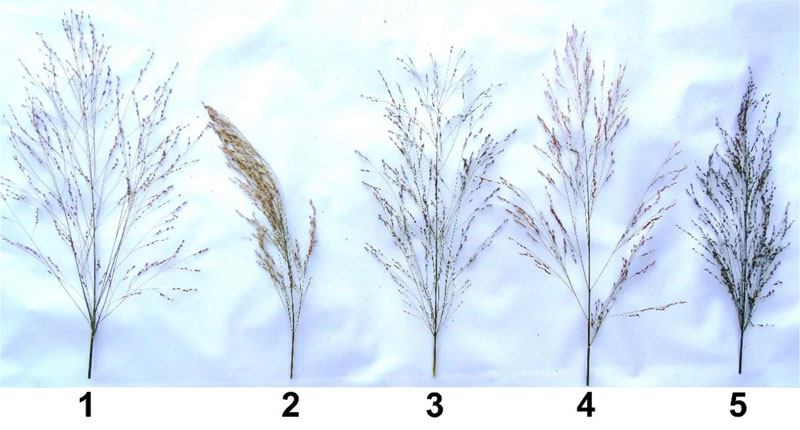
Genotype of P. virgatum | Plant Height, cm | Stem Diameter, mm | Number of Internodes on the Stem | Leaves | ||
---|---|---|---|---|---|---|
Stem Number (per clump) | Length, cm | Width, cm | ||||
f. VP | 208.00±1.09a | 5.15±0.07a | 6.40±0.20b | 7.20±0.20a | 68.30±0.28a | 1.72±0.05a |
f. SL-2 | 189.50±3.98b | 4.90±0.11b | 6.80±0.13a | 7.00±0.33a | 66.78±3.12b | 1.58±0.04c |
f. PP | 206.00±1.71a | 5.06±0.04a | 6.80±0.52a | 7.20±0.2a | 67.30±0.93ab | 1.63±0.03b |
cv. Zoriane | 198.40±2.12b | 4.92±0.7abc | 6.60±0.65ab | 7.00±0.33a | 69.10±0.34a | 1.68±0.07b |
f. RR | 151.00±5.09c | 4.30±0.22c | 4.90±0.23c | 5.20±0.33b | 59.10±0.84c | 1.28±0.05d |
Form, Variety of P. virgatum |
Above-Ground Mass | Stems | Leaves |
---|---|---|---|
f. VR | 41.81c | 18.4c | 23.41c |
f. RB | 41.35c | 18.61c | 22.74c |
f. VP | 46.1b | 19.82b | 26.28b |
cv. Zoriane | 64.16a | 27.59a | 36.57a |
During the first year of growth, plants of switchgrass existed in different phases: from budding to the end of flowering. Early and late-ripening forms provide above ground mass yields of 33-43 t/ha and 41.6-54.2 t/ha, respectively. These yields are higher than reported in other studies [51, 52]. Similar to the above-mentioned studies [51, 52], it is confirmed that biomass yield is mostly comprised of stems (62-72%), while the smallest part of yield is devoted to inflorescences. On average, the aboveground mass of late-ripen plants in the first year of growth had a significantly higher calorie content (4204 kcal/kg) and energy yield per unit area (79.8 Gcal/ha), compared to the early-ripen form (4098 kcal/kg and 61.7 Gcal/ha, respectively). On the second-third years of vegetation, during the fruit ripening period, the yield of the aboveground mass was 41.35-41.81 t/ha for early-ripening plant forms, while late-ripening ones possessed higher productivity rates - 46.10-64.16 t/ha (Table 2). This data suggests that, in general, switchgrass can be considered as a highly productive energy plant [38].
As the development phase progresses, an augmentation in all plant growth and productivity parameters takes place. Compared to the previous period, plants of early-ripening forms showed enhanced productivity during the flowering phase by 4.5-10.8%, while the late-ripening forms generated a more significant increase - 8.1-20.0% (Table 3). As in the previous phase, the highest productivity was identified for cv. Zoriane. Regarding the structure of its aboveground mass, 38.3-39.9% is devoted to stems, 39.0-44.8% - to leaves and only 16.7-21.5% is devoted to inflorescences. Obviously, these patterns are inherent in all genotypes of switchgrass, regardless of growth conditions or genotype. This observation is consistent with the similar results of other researchers [53].
Following further progress of developmental phases to the seeds ripening phase, a significant decrease in plant productivity is observed. Compared to the previous period, this indicator in switchgrass is decreased at least by 31.4% (Table 4) in comparison with the flowering phase (Table 3). Significant differences have been observed between the samples by weight of 10 productive stems and, in general, of an individual plant, which is explained by a high number of productive stems per plant. Both the productivity of the individual plant and the yield of the aboveground mass significantly decreased (by 21.6-49.6%) by the end of the growing season in comparison with the previous growing stage. Such patterns of productivity changes were observed previously for different varieties of switchgrass, irrespectively of the region of cultivation [2, 3, 41, 54]. The aboveground mass yield of late-ripening forms was significantly higher (by 58-155.6%) than a similar parameter of the early-ripening ones. According to Rogers et al. [55], the strong yield potential of Alamo switchgrass opens optimistic opportunities for its use as a dual-purpose grass.
Form, Variety | Mass, g | Structure of Aboveground Mass, % | |||||
---|---|---|---|---|---|---|---|
Above-ground | Leaves | Stems | Inflorescences | Leaves | Stems | Inflorescences | |
f. VR | 93.5d | 38.2d | 37.3d | 18d | 40.9b | 39.9a | 19.2b |
f. RB | 102.5c | 40.0c | 40.5c | 22b | 39.0c | 39.5a | 21.5a |
f. VP | 120.0b | 52.8b | 47.2b | 20c | 44.0a | 39.3a | 16.7c |
cv. Zoriane | 141.8a | 63.5a | 54.3a | 24a | 44.8a | 38.3b | 16.9c |
Form, Variety plant P. virgatum | Productivity of 10 stems | Per Plant |
---|---|---|
f. VR | 46.3d | 185.2d |
f. RB | 55.5c | 222.0c |
f. VP | 87.9b | 334.0b |
cv. Zoriane | 107.9a | 410.0a |
The biochemical composition of switchgrass plants is very important for its use as a raw material for biofuel production [56]. The content of selected biochemical parameters of switchgrass genotypes was identified on different phases of development for different genotypes. The content of dry matter in all genotypes except for f. PL increased during the growing season (from tillering to fruiting) (Fig. 8). For the above-mentioned genotypes, the maximal content of dry matter was observed during the flowering period. During the tillering period, this indicator ranged from 28.62% (f. VP) to 39.13% (f. PP); during the heading period - from 29.72% (f. VP) to 40.93% (f. PP); during the flowering period - from 34.05% (f. RR) to 48.21% (f. PL); and during the fruiting period - from 41.96% (f. SL-1) to 65.28% (f. PL). According to Piłat et al. [57], the dry matter content of two switchgrass varieties was 23.17-24.59% at the vegetative phase, 28.0-28.04% at the beginning of earing, 30.69-31.33% at the beginning of flowering. These results varied depending on the varieties and the stage of growth.
The results of two-way ANOVA showed that all factors under the study made a significant impact on dry matter content (Fig. 8). In particular, the significance of different factors was as follows: for the cultivar F=2.8 at p<0.01, for plant growth stage F=275.9 at p<0.001 and for their interaction – F=2.18 at p=0.004. These findings suggest that dry matter content is significantly changing during the development of P. virgatum. The identified LSDs within the performed two-way ANOVA showed that the significant difference is observed between all the examined genotypes (comparing the content of dry matter in general at all stages of growth), except the genotype pairs VP-DN and PP-PL. The highest content of dry matter was identified during the fruiting stage in biomass of cv Zoriane. Fig. (8) denotes homogenous groups of dry matter content, identified via LSDs calculation (as a part of one-way ANOVA test), regardless of the development stage.
Accumulation of sugars in the aboveground mass of P. virgatum occurred unevenly during the growing season (Fig. 9). During the tillering period, the total sugar content ranged from 4.44% (f. RB) to 9.15% (сv. Zoriane); during the heading period - from 4.83% (f. PP) to 8.17% (сv. Zoriane); during flowering - from 4.11% (f. SL-2) to 6.86% (сv. Zoriane); during the fruiting period - from 3.11% (f. RB) to 8.69% (сv. Zoriane). Hu et al. [56] reported that switchgrass raw biomass contained 3.7% arabinose, 1.3% galactose, 38% glucose, 22.8% xylose, 22.1% lignin, and 3.7% of ash. According to Butkutė et al. [58], carbohydrates composition as well as lignin in dry raw of switchgrass, depended on the stage of growth. According to Piłat et al. [57], the content of water-soluble carbohydrates for two switchgrass varieties was 5.69-6.0% at the vegetative phase, 4.66-4.88% at the beginning of earing and 4.59-5.28% at the beginning of flowering.
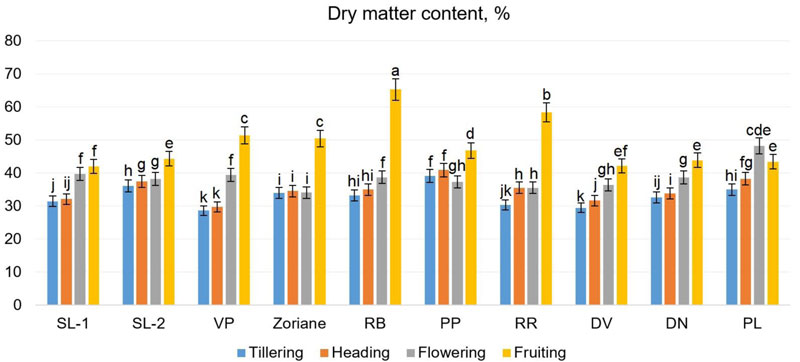
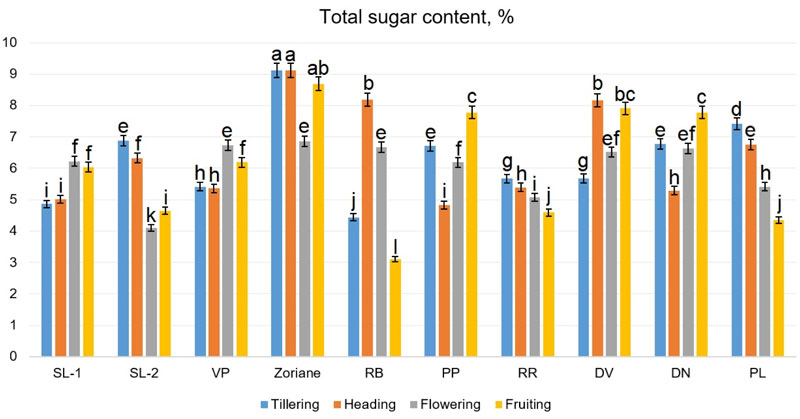
Homogenous groups are identified, regardless of growth stages. Deviation (±) of means is SD.
The two-way ANOVA, conducted for this dataset (Fig. 9), demonstrated a significant influence of all factors, such as genotype (F=695.38 at p<0.001), plant development phase (F=62.13 at p<0.001) and interaction between them (F=300.34 at p<0.001) on the total sugar content values. The determined Fisher’s LSDs with the two-way ANOVA revealed that total sugar content values were significantly different during all growing stages, as they were different for genotypes cv. Zoriane, f. PP, f. RR, f. DV, f. DN. However, the difference was not significant inside genotype groups SL-1-SL-2-RB and VP-PL. The highest content of total sugar was observed in biomass of cv. Zoriane during tillering, heading and fruiting stages, while the lowest ones were identified in f. RB during fruiting. The identified differences in sugar content can be associated with some physiological processes occurring in plants during development, which influence the sugar levels in green mass. Different genotypes had different patterns of change of sugar content during growth, which could be evidence of genotype-dependent individual response. These findings allow us to suggest that plants with a stable or increasing pattern of change in sugar content could be considered as more valuable for further breeding.
In a comparative study of the mineral composition of Miscanthus × giganteus Greef & Deuter, Arundo donax L., Cynara cardunculus L. and P. virgatum L., switchgrass and miscanthus showed the overall better biomass quality than other species [59, 60].
Energy value in the tillering phase ranged from 3964.5 kcal/kg (f. DN) to 4237.7 kcal/kg (f. SL-2); during the ejection of panicles period, from 4004.1 kcal/kg (f. DV) to 4327.3 kcal/kg (f. SL-2); during flowering from 3588.2 kcal/kg (f. PL) to 3719.2 kcal/kg (f. DN); in the period of fruiting from 4102.3 kcal/kg (f. DV) to 4325.4 kcal/kg (f. RB) (Fig. 10). The energy value of switchgrass biomass can reach up to 17 MJ/kg (approximately 4066 kcal/kg), as it was noted by Zhuo et al. (2015) [61] and Giannoulis et al. (2017) [62]. These data are consistent with our results.
The two-way ANOVA test (Fig. 10) revealed that the influence of all factors was significant: genotype factor (F=2.8, p=0.007), growth stage (F=275.87, p<0.001) and interaction of genotype and growth stage factors (F=2.19, p=0.004). Taking into account the calculated LSDs during this test, it has been established that the tested genotypes were very similar by their energy value of biomass. Moreover, the analysis revealed that the difference in energy value was not significant between heading and fruiting stages. To summarise, some correlation can be observed between the energy value and the total sugar content changes: both were decreasing following the development from the heading phase to flowering and slightly increasing during the fruiting stage.
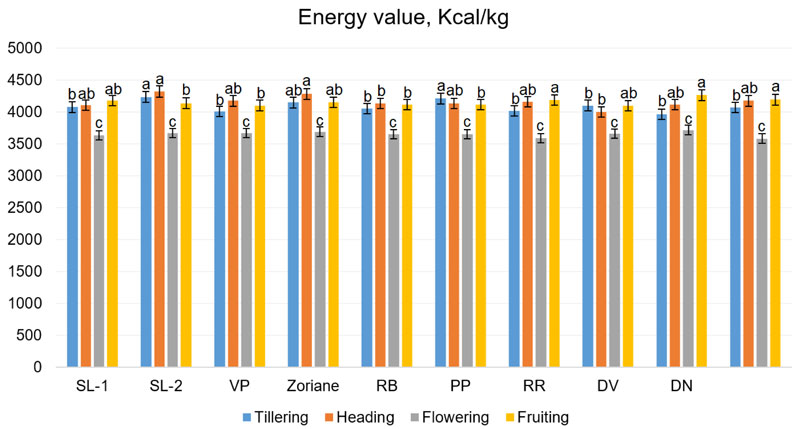
Form, Variety of P. virgatum | Yield of Dry Matter,t/ha | Yield of Ethanol, L/ha |
Energy Yield from Above-Ground Mass, Gcal/ha |
---|---|---|---|
f. VR | 11.84c | 2960b | 51.39b |
f. RB | 11.28c | 2797c | 45.12c |
f. VP | 12.51b | 2989b | 52.85b |
cv. Zoriane | 15.48a | 3669a | 64.94a |
Deviation (±) of means is SD.
As a highly productive culture, P. virgatum provides a high yield of dry matter and, accordingly, energy and ethanol from the aboveground mass at different stages of development. The plant productivity depends essentially on the form and variety characteristics as well as on the phase of development. A sufficiently large yield of dry matter (11.28-15.48 t/ha) and ethanol (2797-3669 l/ha) in various forms of P. virgatum is obtained at the beginning of technical maturity (in the phase of panicle ejection) (Table 5).
Late-ripen forms prevail both in dry matter and ethanol yield. Within the early maturity group, the highest scores were found in VP, while for the late maturity group, it was found in cv. Zoriane. During the heading phase, the tested samples provided a sufficiently great energy yield from the aboveground mass (45.12-64.94 Gcal/ha). The productivity of switchgrass in dry matter, ethanol, and energy of aboveground mass significantly increased during the flowering phase, when compared to the heading phase. Depending on the genotype, the difference in the yield of dry matter was about 29.6-41.7%, of ethanol - 28.1-39.3% and of energy output from the aboveground mass - 35.7-40.9% (Table 6).
A study of American varieties of switchgrass in the Ningxia Province of China showed that cv. Alamo had the highest dry matter with a yield of 44.22 t/ha, cv. Kanlow and Cave-in-Rock had 26.73 and 20.14 t/ha dry matter yield, respectively. The yield of green mass was up to 10.59 t/ha [63]. However, Mantino et al. [64] indicated that the biomass yield of the Alamo variety was 13.3 t/ha and Blackwell variety - 7.5 t/ha in Italy. As shown in Table 6, the yield of the dry mass of some Ukrainian varieties (forms) was higher than the one described above for the American varieties cultivated under the conditions close to those of Italy and less than the same cultivars in China. As reported by Sharma et al. [65], the dry matter yield of P. virgatum cultivars on the third year of their investigation was 12.36 t/ha.
Among the samples tested, the highest dry matter yield (21.7-34.3%), energy (21.4-34.0%), and ethanol (16.2-29.8%) were obtained from the late-ripening group of plants (f. VP and сv. Zoriane). In the respective ripening groups, f. RB and сv. Zoriane showed the highest productivity levels. At the end of the active vegetation period, the main productivity indicators significantly went down. Specifically, in the seed ripening period, the dry matter output decreased by 9.5-40.1%, ethanol – by 11.5-41.3%, energy – by 6.3-37.7% compared to the flowering phase of development (Table 7).
Form, Variety of switchgrass |
Yield of dry matter, t/ha |
Energy yield from above-ground mass, Gcal/ha | Yield of ethanol, L/ha |
---|---|---|---|
F. VR | 14.62c | 61.21d | 3582d |
F. RB | 16.33b | 68.26c | 3936c |
F. VP | 17.79b | 74.29b | 4163b |
cv. Zoriane | 21.93a | 91.49a | 5110a |
Form, variety of switchgrass | Yield of dry matter, t/ha |
Yield of ethanol, L/ha |
Energy yield from above-ground mass, Gcal/ha |
---|---|---|---|
F. VR | 8.76d | 2102d | 38.11d |
F. RB | 11.33c | 2685c | 49.29c |
F. VP | 15.64b | 3613b | 66.78b |
cv. Zoriane | 19.84a | 4524a | 85.71a |
The productivity of late-ripening forms of switchgrass significantly outweighed that of the early forms. Namely, the difference in the dry matter yield in the aboveground mass was 75.1-78.5%, in ethanol 68.5-71.9% and in energy 73.9-75.2%. It should be noted that by the harvest time, the content of dry matter got significantly higher than in the previous stage, while sugar content went dramatically down. That was clearly a manifestation of the vegetative green mass dying.
Among the tested genotypes, сv. Zoriane demonstrated the highest productivity, while f. VR showed the lowest one. In all the studied accessions, the least content of dry matter was in stems (Table 8). In the leaves, it varied from 84.51% (f. SL-2) to 88.52% (f. SL-1); from 48.54% (f. VP) to 55.38% (f. SL-1) in the stems; and from 82.24% (cv. Zoriane) to 87.09% (f. SL-1) in the inflorescences. Hu et al. [56] found that nodes, leaves and internodes of P. virgatum samples contained no significant values of carbohydrates.
The two-way ANOVA analysis conducted for the dry matter content dataset showed that only plant organ factor had a significant influence (F=372.77, p<0.001), while others, including genotype (F=1.55, p=0.2) and genotype-organ factors interaction (F=0.27, p=0.98), were statistically insignificant. These findings indicate that all studied switchgrass genotypes possessed the same dry matter content in different plant organs. However, by the harvesting period, the yield of dry matter would not be determined by this factor. In addition, it was found that during the harvesting period, the stems remained relatively undried and contained 48.54-55.38% of dry matter.
Another two-way ANOVA-test, made for the sugar content dataset, demonstrated that all factors had a significant influence genotype (F=59.83, p<0.001), plant organ (F=150, p<0.001) and their interaction (F=14.9, p<0.001). It was also established that sugar content within leaves and panicles, in general, was not significant. Genotypes SL-1 and PP, as well as Sl-2 and Vp, were not significantly different from each other. The highest content of sugars was found in organs of cv. Zoriane and f. RR.
Switchgrass genotype | Plant organ | Dry matter content, % | Sugar content % |
---|---|---|---|
f. SL-1 | leaves | 88.52±1.58a | 0.49±0.02e |
stems | 55.38±2.45b | 1.20±0.03c | |
panicles | 87.09±3.54a | 0.53±0.05e | |
f. PP | leaves | 88.40±2.44a | 0.53±0.06e |
stems | 54.51±3.11b | 0.86±0.08d | |
panicles | 85.79±4.22a | 1.16±0.07c | |
сv. Zoriane | leaves | 86.21±4.56a | 1.59±0.12b |
stems | 50.74±2.24bc | 2.38±0.21a | |
panicles | 82.24±5.52a | 1.64±0.14b | |
f. VP | leaves | 85.31±2.03a | 0.91±0.06cd |
stems | 48.54±0.57c | 1.96±0.11a | |
panicles | 83.82±4.42a | 0.50±0.02e | |
f. SL-2 | leaves | 84.51±6.32a | 1.08±0.25c |
stems | 52.46±4.13bc | 1.57±0.45b | |
panicles | 85.17±6.22a | 0.51±0.23e | |
f. RR | leaves | 84.99±6.26a | 0.91±0.21cd |
stems | 53.23±0.45b | 2.57±0.11a | |
panicles | 85.92±7.12a | 1.16±0.13c |
It is worth noting that variations in the chemical composition of different switchgrass organs in the present study were lower than those reported in other studies [58]. In our case, the tested varieties of switchgrass contained lower levels of dry matter and sugars in stems (Table 8). Not only for bioenergy purposes, but the investigation of switchgrass raw biomass has also been of interest for agricultural studies (for example, in the USA), due to a realistic possibility of its usage as a forage plant for beef cattle. This research findings show that switchgrass should be harvested for feeding purpose before the maturity stage, when biomass composition is still optimal [60, 66], i.e., for biomass to contain high sugar levels, it has to be harvested right after the fruiting stage, when their content is still high (Fig. 9). Such prospect of P. virgatum cultivation for cattle forage has been supported in a similar study conducted in Mediterranean area which demonstrated that this perennial and high productive plant can serve as a perspective forage crop [64].
The parameters of the energy value of different organs of the studied plants are illustrated in Fig. (11). In leaves, it ranged from 3472.44 kcal/kg (f. VP) to 3904.54 kcal/kg (f. RR); in stems from 3935.32 kcal/kg (f. SL-1) to 4175.28 kcal/kg (f. PP); and in the panicles from 3913.24 kcal/kg (f. SL-2) to 4246.91 kcal/kg (f. RR).
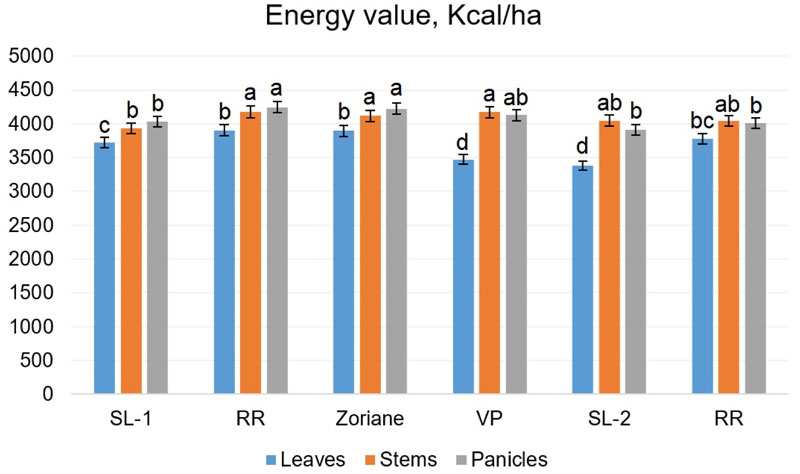
Switchgrass Genotype | The Investigated Organ | Yield of Dry Matter t/ha | Energy Yield from Above-Ground Mass, Gcal/ha |
---|---|---|---|
f. VP | leaves | 8.19e | 28.44d |
stems | 9.36d | 39.06c | |
panicles | 2.58i | 10.65gh | |
Total* | 20.13b* | 78.15c* | |
f. SL-2 | leaves | 7.35f | 24.85e |
stems | 9.56d | 38.70c | |
panicles | 1.96j | 7.67f | |
Total* | 18.87c* | 71.22d* | |
f. PP | leaves | 7.43f | 29.01d |
stems | 10.25c | 42.79b | |
panicles | 2.32i | 9.85h | |
Total* | 20.0b* | 81.65b* | |
сv. Zoriane | leaves | 11.55b | 44.98b |
stems | 12.67a | 52.15a | |
panicles | 3.04h | 12.84g | |
Total* | 27.26a* | 109.97a* | |
f. RR | leaves | 6.31g | 22.54f |
stems | 9.88cd | 40.24bc | |
panicles | 1.30k | 5.14i | |
Total* | 17.49d* | 67.92e* |
The results of conducted two-way ANOVA have shown significant influence of all factors: genotype factor (F=21.13, p<0.001), plant organ (F=149, p<0.001) and interaction of genotype and plant organ factors (F=6.96, p<0.001). Calculated LSDs revealed that the difference between energy values of stems and panicles is not significant, while the energy value of leaves was lower. Similar findings were described by Giannoulis et al. [62], who showed that calorific value in different organs of P. virgatum increased in the following order: leaves, stems, floral stems. The latter was found 17.7 MJ/kg (approximately 4234 kcal/kg). Genotypes were differentiated into three significantly various groups: SL-1-VP-RR, PP-Zoriane and SL-2.
The main productive indices of switchgrass are the output of dry matter, energy and heat capacity of the raw material, which depend on the aboveground organs of the plants (Table 9). The stems provide the highest yield of dry matter and energy per unit area, and the lowest rates are in the panicles. In most specimens, the stems were marked by the heat capacity and in the individual specimens in the panicles. Conducted two-way ANOVA for data on biomass yield and energy output (per ha) from different plant organs (Table 9) has shown that influence of all factors and their interactions was statistically significant, which was to be expected. Stems and leaves contributed significantly greater amounts to the total biomass yields than panicles. Additionally, stems provided the vast majority of energy output (per ha), since they possessed a higher calorific value than leaves, and provided more green mass.
Genomics associated breeding of switchgrass can help improve the development of high-productive genotypes of this species in the future [67, 68]. Summarizing the obtained results, one of the most important germplasm collections for the introduction and breeding of switchgrass in Ukraine and, probably, in the whole Eastern European region has been created in M.M. Gryshko NBG (Kyiv). This institution has collected a valuable gene pool of plants from the Americas, Europe, and Asia. With the use of traditional breeding techniques, a number of breeding forms and varieties of switchgrass have been developed and successfully approbated in different experimental areas, including cultivation and processing technologies. The obtained genotypes of P. virgatum are not inferior to other known perennial energy plants. The results of our research demonstrate the success of introducing switchgrass plants under the climatic conditions of the Forest-Steppe region of Eastern Europe, including Ukraine.
CONCLUSION
Comprehensive studies have been conducted to estimate the morphological, productive and ecological traits, as well as the biochemical composition of 10 switchgrass (Panicum virgatum L.) genotypes from the collection of 33 switchgrass accessions at M.M. Gryshko NBG. The investigated breeding lines and a variety have been introduced, acclimated and developed as high-yielding genotypes suitable for growing in the Ukrainian Forest-Steppe zone. It has been shown that switchgrass undergoes a complete life cycle without abnormalities of developmental stages, starting from the first year of growth. Three types of plants have been described according to their life cycle length: early-ripening (produce seeds by the beginning of September), mid-term-ripening (by the end of September) and late-ripening forms (by mid-October), which are able to tolerate moderate frosts. Additionally, it has been established that the late-ripening form of switchgrass exceeds early-ripening ones by most of the morphometric parameters, which predetermine their high productivity. The biggest difference in morphology between the genotypes has been observed in the third year of growth. It has also been shown that some genotypes (including cv. Zoriane) are able to produce more biomass than the widely-known variety Alamo.
The vast majority of the studied switchgrass genotypes accumulated dry matter during the growing season, including the fruiting phase. The sugars in the biomass were accumulating unevenly throughout the vegetative period: for most of the genotypes, a decrease in sugar content during the flowering phase was observed. Switchgrass productivity by the yield of dry matter from the aboveground mass during the flowering phase had significantly increased compared to the heading phase. By the end of the active vegetation period of plants, the main productive indicators decreased. The largest proportion in the structure of the aboveground mass was accounted for stems, while the smallest - for the inflorescences. The main productive parameters of switchgrass on the yield, such as dry matter, energy value and heat capacity of the raw material, were highly dependent on the aboveground organs of the plants. The prevailing organs in terms of dry matter and energy yield per unit of the area were stems. The lowest energy value, when evaluated between panicles, stems and leaves, has been determined for leaves. Apart from the evaluation of biomass yield and energy output, the possibility of generating second-generation bioethanol has been successfully tested, as well as potential ethanol yield per area unit was estimated (for some genotypes). The most productive lines have been identified, among which special interest possessed cultivar Zoriane. As a result of all conducted breeding investigations, a valuable pool of high-productive switchgrass genotypes has been created, which are suitable for biofuels and amenable for cultivation under the climatic conditions of the Forest-Steppe region of Eastern Europe, including Ukraine.
ETHICS APPROVAL AND CONSENT TO PARTICIPATE
Not applicable.
HUMAN AND ANIMAL RIGHTS
No animals/humans were used for studies that are the basis of this research.
CONSENT FOR PUBLICATION
Not applicable.
AVAILABILITY OF DATA AND MATERIALS
The data supporting the findings of the article was not reposited. All the data that the authors intended to share is in the present article.
FUNDING
This research was carried out within the frameworks of R&D project “Selection and creation of high-yielding genotypes of energy plants - potential sources of second generation bioethanol" (State registration N 0113U002925) of R&D Programme of Natl. Academy of Sci. of Ukraine “Biological Resources and New Technologies of Bioconversion” (2013-17).
CONFLICT OF INTEREST
The authors declare no conflict of interest, financial or otherwise.
ACKNOWLEDGEMENTS
We acknowledge the suggestions by Dr. V. Kyrylenko (Institute of Food Biotechnology and Genomics, National Academy of Sciences of Ukraine, Kyiv) aimed to improve the manuscript language.