All published articles of this journal are available on ScienceDirect.
Influence of Nitrogen Application and Drip Irrigation on Greenhouse Gas Emissions and Yield of Cardoon Crop (Cynara cardunculus L.)
Abstract
Background:
Cardoon is a multi-purpose crop with a wide spectrum of potential applications.
Objective:
The aim of this study was to assess the effects of nitrogen (N) application and drip irrigation practices on emissions of nitrous oxide (N2O), carbon dioxide (CO2) and methane (CH4) and morphological characterization and biomass production of cardoon crop.
Methods:
The experiment was run under field conditions from April to August 2018. The field experiment was a randomized complete block design with three replications and four treatments: (i) non-amended soil as control (CONTR); (ii) mineral N fertilizer and non-irrigated (NITRO); (iii) non-fertilized and drip irrigation (IRRIG); and (iv) mineral N fertilizer and drip irrigation by fertigation (FERTI). The fluxes of N2O, CO2 and CH4 were measured by the closed chamber technique and the morphological characteristics and yield of cardoon plants were evaluated per treatment.
Results:
Results showed that the N2O emissions and global warming potential were not significantly different among the application of mineral N fertilizer itself or by drip fertigation with an emission rate of 0.5% of N applied and -6,8 t CO2-eq. ha-1. The characteristics related to biomass production did not differ significantly (p>0.05) between amended treatments although numerically higher in IRRIG treatment.
Conclusion:
It was concluded that the application of mineral N fertilizer itself or drip fertigation had no significant effect on N2O emissions and global warming potential. Additionally, the plant growth, including biomass production, increased due to drip irrigation, but not significantly.
1. INTRODUCTION
Cardoon is an herbaceous perennial species native to the Mediterranean basin, with a cross-pollinated system and a highly heterozygous genetic background. Cardoon is a multi-purpose and versatile crop with a large variety of potential applications, due to its high added value biomass as a rich source of bioactive compounds [1]. Cardoon species are a component of the Mediterranean diet and have been used for food and medicine since ancient times. The numerous pharmacological actions reported include studies related to hepatoprotective, diuretic, choleretic, antioxidant, anticarcinogenic, anticholestatic, anti-influenza, antiviral, antimicrobial, antifungal, anti-HIV, anticholesterol, low-density lipoprotein oxidation and anti-inflammatory activities [2]. However, the content of bioactive compounds in plants depends on several factors including the edaphoclimatic conditions, genetics, type of plant tissues, growth stage and extraction procedures [3], as well as on management practices including fertilization programs [4, 5].
Cardoon can also be exploited for dry biomass production and energy purposes, raw material for paper pulp and lignocellulosic biomass for lightwood panels [3]. Achenes can be used not only for biofuel production and bioplastics but also for human consumption due to its high nutritional value [6]. It can be used in ruminant nutrition as green forage or preserved silage, with high organic matter digestibility [7]. Thus, these characteristics, among others, enable the development of innovative products in food and nutrition, pharmaceuticals and cosmetics, plant protection and biocides, and healthcare industries [8]. Particularly, in Mediterranean regions, aqueous extracts from cardoon flowers have been used for centuries as a coagulant in ewes milk cheese traditional manufacture. Due to their high content of aspartic proteases with milk-clotting activity, the cardoon extracts generate specific characteristics of texture and flavour on cheese. According to the national regulation of Serra da Estrela cheese, the use of cardoon flowers as a coagulant is mandatory and has been recognized as a key factor in cheese authenticity [9].
Cardoon is a plant well adapted to the Mediterranean climatic conditions where rainfall is low, irregular and mainly concentrated in autumn/winter (450-1000 mm year-1) and the air temperature range between 7-38 ºC [1]. The soils with levels of pH 5.0-8.6, soil salinity of 4-10 dS cm-1 and 50-150 cm soil depth, are considered suitable for cardoon growth. Cardoon, considered tolerant of poor soils, can be suggested as a potential alternative crop for conserving the fragile agroecosystems with low fertility and with positive effects on soil water management and erosion control [10]. In fact, its perennial life form, annual growth cycle and adaptation to drought stress make this plant a superior candidate to be grown in the dry and marginal lands of the Mediterranean region as a field crop for multipurpose uses [3, 8, 9]. Therefore, the cultivation of cardoon, established from seed or by nursery seedlings [10], as a non-conventional field crop by the agricultural sector is in line with the requirement for adapted and sustainable crops in the context of the forthcoming climatic changes with increasingly severe droughts. However, the use of cardoon as a field crop has only recently been compared with other crops and little is known about the application effects of agronomic practices such as fertilization. Nitrogen (N) by far is the most needed mineral nutrient in agroecosystems [11, 12], and agricultural crops have a general response to N fertilizers application while this is not the case for other mineral nutrients [13, 14]. Despite that previous authors [3, 15, 16] conducted studies on N fertilization of the cardoon crop; however, optimal fertilizer rates that balance crop yield, quality and sustainability are not yet completely established. In addition, an N fertilization rate between 0 and 160 kg N ha-1 had a positive effect on biomass and seed yield of cardoon on a fertile soil [15] whereas an N fertilization rate between 50 and 200 kg N ha-1 together with irrigation had no influence on yield of aboveground biomass [16].
Therefore, the aim of this study was to evaluate the effects of N application and drip irrigation practices on the emission of nitrous oxide (N2O), carbon dioxide (CO2) and methane (CH4) and morphological characteristics and yield of cardoon crop.
2. MATERIALS AND METHODS
2.1. Experimental Site
The experiment was run under field conditions in a Dystric Cambisol [17], from April to August 2018. On May 2017, the cardoon plants (Cynara cardunculus L. var. altilis DC.) were installed in an agricultural field located at Associação Portuguesa de Pais e Amigos do Cidadão Deficiente Mental (APPACDM) of Viseu, Portugal (latitude: 40º37´49´´N, longitude: 7º55´48´´W) (Fig. 1). The physico-chemical properties of the agricultural soil (0-500 mm) were characterized by standard analytical methods [18-22] and are given in Table 1.
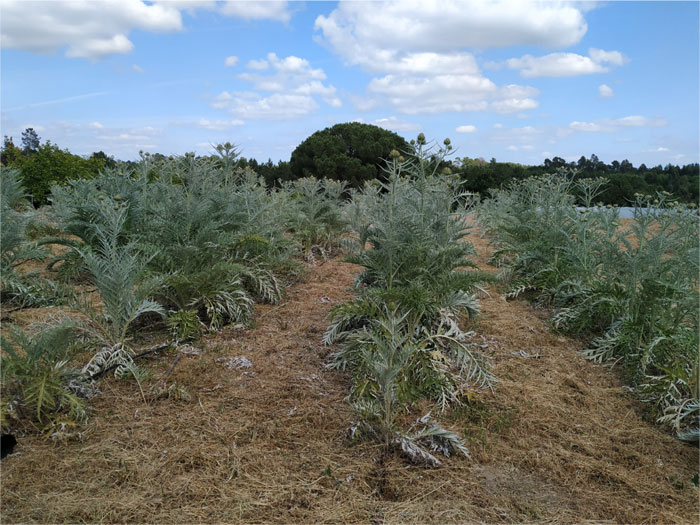
Parameter | Value | Method |
---|---|---|
Soil classification | Dystric Cambisol | World Reference Base |
Texture | loamy-sand | International pipette |
Coarse sand (0.2-2 mm) | 462±7 g kg-1 | International pipette |
Fine sand (0.02-0.2 mm) | 150±17 g kg-1 | International pipette |
Silt (0.002-0.02 mm) | 258±12 g kg-1 | International pipette |
Clay (<0.002 mm) | 130±2 g kg-1 | International pipette |
Bulk density | 1.1±0.1 g cm-3 | Keen & Raczkowski method |
pH (H2O) | 4.4±0.1 | EN 13037 method |
Water retention capacity at pF 2.0 | 336.4±3.5 g kg-1 | Gravimetric method |
Organic matter | 22.2±0.3 g kg-1 dry soil | Dumas method |
Extractable P | 274±16 mg kg-1 dry soil | Enger-Riehm method |
Extractable K | 208±13 mg kg-1 dry soil | Enger-Riehm method |
Effective cation exchange capacity | 8.4±0.1 cmol(+) kg-1 | Ammonium acetate method |
Month | Air Temperature | Relative Humidity | Wind Velocity | Cumulative Rainfall | Cumulative Irrigation |
---|---|---|---|---|---|
(ºC) | (%) | (m s-1) | (mm) | (mm) | |
April 2018 | 11.4±3.5 | 83.5±3.4 | 3.8±0.2 | 152 | 70 |
May 2018 | 14.4±4.1 | 76.3±5.5 | 3.9±0.4 | 32 | 100 |
June 2018 | 17.4±3.7 | 87.9±6.5 | 3.5±0.3 | 91 | 100 |
July 2018 | 19.3±4.2 | 87.9±1.6 | 3.0±0.1 | 11 | 100 |
August 2018 | 24.2±5.1 | 60.8±0.7 | 4.0±0.3 | 1 | 90 |
This region has a Mediterranean influence with an annual rainfall of about 1200 mm (76% rainfall between October and March) and a mean annual temperature of 14.0 ºC (mean temperatures of 21.7 ºC in the warmest month and 7.1 ºC in the coldest month). Usually, the agricultural crops are irrigated in the spring/summer seasons (April to September) and rainfed in the autumn/winter seasons. An automatic compact weather station (WS-GP1, Delta-T Devices Ltd, UK) located near the experimental site was used to collect climatic data during the experimental period (Table 2).
2.2. Treatments Application
The experiment was a randomized complete block design with three replicates and four treatments. The treatments considered were:
(1) Non-amended soil and without irrigation as control (CONTR treatment);
(2) Application of mineral N as basal fertilizer and without irrigation (NITRO treatment);
(3) Non-fertilized and drip irrigation during the growing season (IRRIG treatment);
(4) Application of mineral N dissolved in irrigation water and split by drip irrigation as fertigation (FERTI treatment).
Each plot had an area of 60 m2 (10 m width × 6 m length), with 2 m between plots and a density of 10000 plants ha-1. On April 13, the NITRO treatment received, directly to the soil surface, one application of mineral fertilizer at a rate of 25 kg N ha-1 (ammonium nitrate (NH4NO3)). Then, it was sprayed homogenously and mixed into the upper soil (0-50 mm) using a hoe. From April 13 to August 27, the IRRIG treatment received a total of 460 mm of water by drip irrigation using short irrigation events of 10 mm every 3 days (30% water-filled pore space (WFPS)). For the FERTI treatment, mineral N at a rate of 25 kg N ha-1 (NH4NO3) was dissolved in irrigation water (total=460 mm) and split into short irrigation events of 10 mm every 3 days, similar to those in IRRIG treatment. On April 13, all plots received the same tillage practice of NITRO treatment.
2.3. Greenhouse Gas Fluxes
Fluxes of N2O, CO2 and CH4 were measured by the closed chamber technique [18, 23, 24]. Gas measurements were carried out daily during the first 7 days, every 3 days in the next two weeks and then once a week until the end of the experiment. For evaluating the gas fluxes from each treatment, a circular chamber of polyvinyl chloride (Ø=200 mm, h=110 mm), equipped with a septum to sample the interior atmosphere, was inserted into the soil (depth=30 mm). The chambers were kept at fixed locations throughout the sampling dates. After the chamber was closed, a first gas sample (25 mL) was taken (t=0 min) using a plastic syringe and flushed through gas vials (20 mL) and then a second gas sample was taken (t=60 min) from the headspace of the chamber and stored in vials.
The gas samples were analyzed by gas chromatography (GC-2014, Shimadzu, Japan) with the following detectors: electron capture 63Ni detector for N2O (accuracy=50 ppb to 100 ppm), thermal conductivity detector for CO2 (accuracy=1 ppm to 1%) and flame ionization detector for CH4 (accuracy=0.1 ppm to 1%). The N2O, CO2 and CH4 fluxes were determinate by the Eq. (1).
![]() |
(1) |
Where FLU was the N2O, CH4 or CO2 flux on each sampling date (FLU, g N or C m-2 day-1), CON was the gas concentration (m3 m-3 min-1), MOL was the gas molecular weight (44 g mol-1 for N2O or CO2 and 16 g mol-1 for CH4), IDE was the volume of an ideal gas (0.0224 m3 mol-1), TEM was the temperature during the sampling period (ºC), HEI was the height of the chamber (0.080 m) and TIM was the time corrected for a 1-day duration (1440 min).
The cumulative gas emissions were estimated by averaging the flux between two sampling occasions and multiplying by the time interval between the measurements. The conversion factors of 265 for N2O and 28 for CH4 were used to express the Global Warming Potential (GWP) as CO2-equivalents [18, 23, 24].
2.4. Cardoon Productivity
The morphological characteristics of cardoon plants were evaluated from July to August 2018, based on international union for the protection of new varieties of plant descriptors [9, 24], being considered the following parameters: Plant Height (PH), Number of Stems (NS), Number of Primary Ramifications (NPR) and Number of Secondary Ramifications (NSR), Number of Inflorescences (NI), Stem Diameter at the Base (SDB) and at the Top (SDT).
In order to evaluate biomass production at the harvest (August 27), all plant materials were collected separately, immediately weighed and oven-dried at 65 ºC (until a constant weight was reached) to determine the dry matter content of each plant component: Leaves Dry Weight (LDW), Stems Dry Weight (SDW), Inflorescences Dry Weight (IDW), Total Plant Dry Weight (TPDW), Total Flowers (TFDW), Total Achens Dry Weight (TADW) and Achenes Yield in the Inflorescences Dry Weight (AYIDW).
2.5. Statistical Analysis
Greenhouse gas emissions, morphological characteristics and biomass production were evaluated by analysis of variance (randomized complete block design) to test the effect of treatments (CONTR, NITRO, IRRIG and FERTI). The statistical significance (p<0.05) of the mean differences between treatments was determined by the Tukey Honestly Significant Difference test. The statistical software package used was STATISTIX 10 (Analytical software, Tallahassee, FL, USA).
3. RESULTS AND DISCUSSION
3.1. Greenhouse Gas Emissions
The daily fluxes of N2O, CO2 and CH4 from treatments are shown in Fig. (2). A significant increase (p<0.05) of N2O fluxes of amended treatments relative to CONTR treatment was observed during the daily measurements and a first peak was observed on April 13 (114-172 µg N2O-N m-2 day-1) (Fig. 2A). Then, the N2O fluxes decreased in all treatments followed by a second peak on May 14 (212-310 µg N2O-N m-2 day-1) and a third peak on 18 July (222-245 µg N2O-N m-2 day-1) (Fig. 2A). In the NITRO and FERTI treatments, N2O fluxes were generally higher than in IRRIG treatment, spite the differences were not statistically significant (p>0.05) (Fig. 2A). Cumulative N2O emissions from amended treatments were significantly higher (p<0.05) than in the CONTR treatment (three times higher in NITRO and FERTI treatments and one time higher in IRRIG treatment) (Table 3). Thus, the N2O cumulative emissions did not differ significantly (p>0.05) between NITRO and FERTI treatments but were significantly higher (p<0.05) in ca. 100% relative to IRRIG treatment (Table 3). Previous studies [25-27] reported that the application between 60 and 125 kg ha-1 of inorganic N led to an increase of N2O emissions mainly by nitrification and/or denitrification processes, depending on the soil water content, even though nitrifier denitrification can be also a relevant source of this gas, especially under certain soil conditions. Additionally, the increase of the soil WFPS by drip irrigation events have a significant impact on nitrification and denitrification processes [28-30]. Teira-Esmatges et al. [31] reported that nitrification was the dominant source of N2O in a 30-70% WFPS range. Thus, nitrification may be the major process of N2O losses in NITRO treatment, but denitrification in the micropores should also be considered in IRRIG and FERTI treatments. In addition, the N2O cumulative emissions, expressed as a percentage of N applied, were not significantly different (p>0.05) between NITRO and FERTI treatments, representing an average emission rate of 0.5% of N applied (Table 3). The results of this study are in line with Cayuela et al. [32] who suggest an average N2O emission rate of 0.5% of N input for Mediterranean crops.
Treatments | N2O (g N ha-1) |
N2O (% N applied) |
CO2 (kg ha-1) |
CH4 (kg ha-1) |
GWP (kg CO2-eq. ha-1) |
---|---|---|---|---|---|
CONTR | 29±9c | 9867±475ab | -553±181ab | -5623±4675ab | |
NITRO | 126±66a | 0.50±0.26a | 12885±1002a | -809±250a | -9723±7747a |
IRRIG | 62±15b | 9886±882ab | -461±240a | -3010±5910a | |
FERTI | 119±19a | 0.48±0.08a | 7790±459b | -556±93ab | -7750±2395ab |
Values presented with different superscripts within columns are significantly different (p<0.05) by Tukey test.
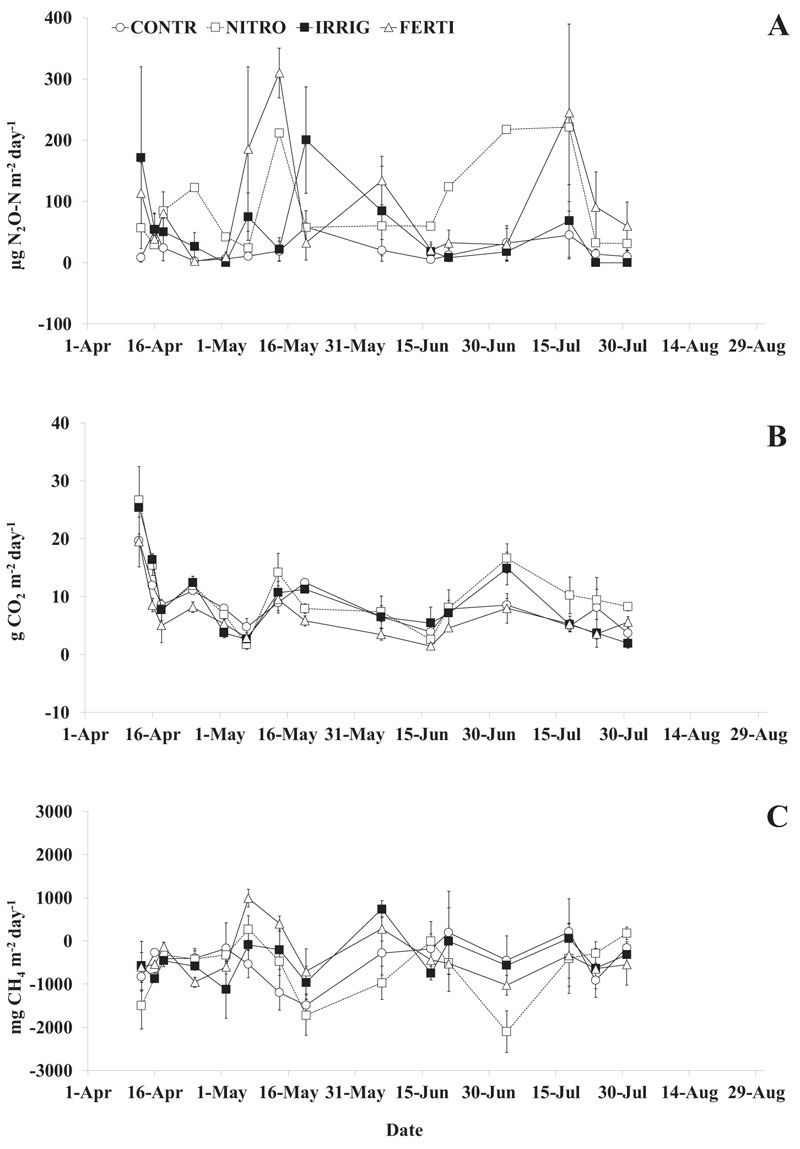
The CO2 daily fluxes peaked in all treatments immediately after the beginning of the experiment (19-25 g CO2 m-2 day-1 on April 14) followed by a second peak on July 4 (8-17 g CO2 m-2 day-1) (Fig. 2B). The CO2 daily fluxes from NITRO treatment were higher in most measurement dates relative to all other treatments, even if differences were not always significant (p>0.05) (Fig. 2B). The CO2 cumulative emissions present no significant differences (p>0.05) between CONTR and IRRIG treatments (Table 3). However, the same variable from NITRO treatment was significantly higher (p<0.05) in ca. 65% relative to FERTI treatment (Table 3). The application of inorganic N could increase, decrease, or maintain the CO2 fluxes from soils whereas the incorporation of crop residues enhances the carbon mineralization [33-36]. In the present study, the application of N fertilizer could have increased microbial activity [37] and then increased soil respiration, while the irrigation/rainfall could have reduced the availability of N fertilizer.
The CH4 daily fluxes varied from negative to positive values (-2107 to 988 mg CH4 m-2 day-1) in all treatments throughout the measurement period (Fig. 2C). Thus, the CH4 daily fluxes were mainly negative, except in IRRIG and FERTI treatments with positive values (279-988 mg CH4 m-2 day-1) in some measurement dates (May 7-14 and June 6) (Fig. 2C). The CH4 soil emissions result from the balance between anaerobic methanogenic CH4 production and aerobic methanotrophic CH4 consumption, being controlled by soil aeration [29]. Usually, the well-drained agricultural soils act as CH4 sink. The rainfall between April and June as well as the drip irrigation events should have enhanced the methanogenic activity, resulting in net CH4 emission [26]. However, the CH4 cumulative emissions as well as the GWP did not differ significantly (p>0.05) among treatments, with an average value of about -600 kg CH4 ha-1 and -6,8 t CO2 eq. ha-1, respectively (Table 3).
3.2. Cardoon Productivity
The morphological characterization and biomass production of cardoon plants per treatment are presented in Tables 4 and 5. On average, plant height was 1561 mm, with the lowest primary ramification obtained at 960 mm and the upper ramification at 1206 mm from the soil. The stalk diameter has an average of 23.7 mm at the base and 7.7 mm at the top. The average number of ramifications on the main stem was discriminated on primary and secondary ramifications with 3.8 and 6.7, respectively. The total number of inflorescences was 17.2, being split in each plant by 2 main inflorescences, 6 primary inflorescences and 9 secondary inflorescences. The length and diameter of the principal inflorescences were lower than secondary inflorescences (61.5 against 51.1 mm for length and 67.2 against 51.5 mm for diameter). The average total production of flowers and seeds per plant was 42.5 g and 77.6 g, respectively. The total average seed weight by inflorescence was 4.5 g; however, the value varied between 8.4 g in the main capitula, 7.5 g and 3.5 g in primary and secondary inflorescences, respectively. The average individual seed weight in the principal, primary and secondary inflorescences was 0.048 g, 0.045 g and 0.041 g, respectively. The seed weight per plant was 77.6 g which represents 13.9% of the inflorescence weight. Each capitulum produced 2.6 g of flowers and 4.4 g of seeds. The flowers harvesting occurred with a frequency of 3-5 days between June 29 till August 10 with a total production of 5338 g. The total seed production was also 7470 g.
The Plant Height (PH) in IRRIG treatment was significantly higher (p<0.05) in about 20% relative to FERTI treatment (Table 4). The Height of Lower Branch (HLB) and Upper Branch (HUB) did not differ significantly (p>0.05) among treatments, although being higher in CONTR treatment and lower in FERTI treatment (Table 4). The number of secondary ramifications (NSR) from the CONTR treatment was significantly higher (p<0.05) in 100% relative to FERTI treatment and 40% than NITRO or IRRIG treatments (Table 4). The number of stems and primary ramifications did not differ significantly (p>0.05) between treatments (Table 4). The CONTR treatment presented the highest number of secondary ramifications and inflorescences followed by IRRIG and the FERTI treatment revealed the lowest number (Table 4). The plants of CONTR treatment showed the lowest height of the first ramification and the highest stem diameter at the base which promoted plants with the highest number of inflorescences (Table 4). The distance between the Lower Branch (HLB) and Upper Branch (HUB) seems to be related with the Number of Secondary Ramifications (NSR) and consequently with the Number of Inflorescences (NI) (Table 4).
The characteristics related with biomass production, namely Leaves Dry Weight (LDW), Stems Dry Weight (SDW), Inflorescences Dry Weight (IDW), Total Plant Dry Weight (TPDW), Total Flowers Dry Weight (TFDW), Total Achenes Dry Weight (TADW), Achenes Yield in the Inflorescences Dry Weight (AYIDW), did not differ significantly (p>0.05) between treatments although numerically higher in IRRIG treatment (Table 5). The CONTR treatment also presented the highest flower production and achenes weight followed by the IRRIG treatment (Table 5). These results are related to the highest number and the largest dimension of inflorescences (Table 5). The plants of IRRIG treatment showed the inflorescences with the highest value of achenes yield (Table 5).
Treatments | PH | NS | NPR | NSR | NI | TNI | HLB | HUB | SDB | SDT | IL | ID |
---|---|---|---|---|---|---|---|---|---|---|---|---|
CONTR | 1573±136ab | 2.0±1.0a | 7.0±3.4a | 13.9±6.3a | 2.0±1.0ab | 23.0±10.2a | 864±9a | 1161±12a | 24.4±2.3a | 8.3±0.6a | 66.4±10.2a | 65.8±15.2a |
NITRO | 1546±104ab | 1.9±0.4a | 6.0±1.3a | 8.4±2.7ab | 4.2±0.4a | 16.3±3.9ab | 936±13a | 1184±9a | 21.7±2.8a | 7.3±0.6a | 59.5±3.9a | 67.2±5.7a |
IRRIG | 1665±115a | 2.0±0.8a | 6.8±2.9a | 7.4±2.8b | 1.3±0.8b | 16.2±6.0ab | 1032±10a | 1298±10a | 22.6±2.8a | 7.6±0.4a | 58.0±6.0a | 70.2±13.8a |
FERTI | 1416±157b | 2.0±0.7a | 5.8±2.0a | 5.8±4.4b | 2.3±0.5ab | 13.4±5.8b | 964±15a | 1164±15a | 24.3±4.0a | 7.5±0.6a | 56.9±5.8a | 64.7±17.9a |
Values presented with different superscripts within columns, are significantly different (p<0.05) by Tukey test.
Treatments | LDW | SDW | IDW | TPDW | TFDW | TADW | AYIDW |
---|---|---|---|---|---|---|---|
CONTR | 77.7±24.6a | 535.9±57.2a | 629.1±38.8a | 1246.1±126.5a | 55.4±15.2a | 83.9±21.5a | 13.8±4.7ab |
NITRO | 77.1±18.2a | 405.7±40.9a | 480.5±35.9a | 963.3±51.1a | 30.8±5.7b | 58.4±3.4a | 12.6±4.3b |
IRRIG | 88.6±31.1a | 462.3±51.3a | 491.6±16.0a | 1042.5±65.6a | 41.8±13.8ab | 81.4±8.8a | 17.4±4.3a |
FERTI | 70.0±21.2a | 423.2±79.1a | 426.4±38.0a | 919.5±70.1a | 35.5±17.9ab | 61.5±4.6a | 11.7±5.6b |
Values presented with different superscripts within columns are significantly different (p<0.05) by Tukey test.
Cardoon biomass production varies considerably with land topography, growing, cultivation management as well as by the genotype [38, 39], being reported a biomass yield that ranges from 1400 to 13700 kg ha-1 for Mediterranean climate conditions [10, 40]. In Spain, Fernández et al. [1] reported an average partitioning of cardoon was 35.4% basal leaves, 13% cauline leaves, 18.7% stems and 32.9% heads; seeds represented 8-10% of the aboveground biomass (all expressed by dry weight). In the present study, the expected total biomass production per hectare was 10400 kg, namely 4600 kg of stems, 5000 kg of inflorescences and 800 kg of leaves. The plants also produced 425 kg of flowers and 750 kg of seeds. These values are according to data obtained in plants of a second-year growth cycle [9, 24]. The weight of leaves obtained in this study (816 kg) was considerably lower compared with the potential if all leaves were collected (4330 kg). In fact, the weight of flowers collected could vary with the intensity of harvesting. The estimated production of the seeds was 750 kg and could vary significantly between fields and depends on plant genotype. Seed harvesting should be done before the dissemination process when the inflorescences are mature. The seed yield obtained from the inflorescences corresponds to 14%, which is below the values reported by other reference studies (25.5-28.7%) [3, 6]. To maximize seed production, flower harvesting should be done 2-3 days after blossom.
CONCLUSION
The present study showed that the application of mineral N fertilizer itself or by drip fertigation had no significant effect on N2O emissions and global warming potential. The results also showed that the plant did not respond well to fertilization, indicating a very good opportunity toward organic production and reduction in mineral fertilizer application in cropping systems. Additionally, plant growth and biomass production increased due to drip irrigation, but not significantly. Nevertheless, long-term field studies are suggested to confirm those results and to evaluate different agricultural practices to maximize or modulate cardoon crop production.
LIST OF ABBREVIATIONS
AYIDW | = Achenes Yield in the Inflorescences Dry Weight (g). |
GHG | = Greenhouse Gases |
GWP | = Global Warming Potential |
HLB | = Height of Lower Branch (mm) |
HUB | = Height of Upper Branch (mm) |
ID | = Inflorescence Diameter (mm) |
IDW | = Inflorescences Dry Weight (g) |
IL | = Inflorescence Length (mm) |
LDW | = Leaves Dry Weight (g) |
NI | = Number of Inflorescences |
NPR | = Number of Primary Ramifications |
NS | = Number of Stems |
NSR | = Number of Secondary Ramifications |
PH | = Plant Height (mm) |
SDB | = Stem Diameter at the Base (mm) |
SDT | = Stem Diameter at the Top (mm) |
SDW | = Stems Dry Weight (g) |
TPDW | = Total Plant Dry Weight (g) |
TADW | = Total Achenes Dry Weight (g) |
TFDW | = Total Flowers Dry Weight (g) |
TNI | = Total Number of Inflorescences |
WFPS | = Water Filled Pore Space |
ETHICS APPROVAL AND CONSENT TO PARTICIPATE
Not applicable.
HUMAN AND ANIMAL RIGHTS
No animals/humans were used for studies that are the basis of this research.
CONSENT FOR PUBLICATION
Not applicable.
AVAILABILITY OF DATA AND MATERIALS
The data supporting the findings of this study are available within the article.
FUNDING
This work was supported by National Funds by FCT - Portuguese Foundation for Science and Technology, under the project UIDB/04033/2020, and projects WASTE2VALUE PDR2020-1.0.1-FEADER-032314 and WASTECLEAN PROJ/IPV/ID&I/019.
CONFLICT OF INTEREST
The authors declare no conflict of interest, financial or otherwise.
ACKNOWLEDGEMENTS
The authors are grateful to Mr. António Figueiredo and the committee of APPACDM (Viseu, Portugal) for all the support to develop this work, as well as the Reviewers for their comments and suggestions.