All published articles of this journal are available on ScienceDirect.
Induction of Bacterial Canker Resistance in Tomato Plants Using Plant Growth Promoting Rhizobacteria
Abstract
Background:
By inducing the production of inhibitory allelochemicals and mechanisms of systemic resistance Plant Growth Promoting Bacteria (PGPB) help plants to cope with stresses.
Materials and Methods:
In this study cell suspensions of Bacillus subtilis, Pseudomonas fluorescens or Azotobacter chroococcum were used to test the efficacy of these PGPB in inducing resistance in tomato (Lycopersicon esculentum Mill) against Clavibacter michiganensis subsp michiganensis, a bacteria known to cause canker disease. To test this hypothesis, seedlings of Chaika variety, characterized by short growing, early-ripening, high productivity and resistance against fusarium and the C. michiganensis strain ІZ-38 isolated in Kyiv were employed.
Results and Conclusion:
The use of cell suspensions of the PGPB B. subtilis, A. chroococcum or P. fluorescens induced an increment in the resistance of tomato plants against the causative agent of bacterial canker (C. michiganensis subsp. michiganensis) by 42–50%. PGPB in fact promoted in C. michiganensis infected tomato plants: i) the accumulation of chlorophyll a and b and carotenoids; ii) the thickening of the upper and lower epidermis of leaves; iii) the deposition of biopolymers with protective properties in epidermal cells; iv) the activity of the peroxidase enzyme and v) the net productivity of photosynthesis.
1. INTRODUCTION
Bacterial canker of tomatoes is a significant problem for farmers and private households [1]. The pathogen enters the plant through the wound surface, hydatodes, stomata and roots where it moves through the phloem, inhabits the bark, the epithelial tissue and pith of the stem [2]. Bacteria multiply in xylem vessels and form biofilm structures that accelerate colonization and movement of pathogens in plants [3]. Through the vessels, the bacteria enter the fruits and predetermine their internal lesions. In particular, bacteria infect the surface of the seeds and penetrate the seed peel, where they are stored and can serve as a source of new infection [4].
Plant infection with bacterial pathogens leads to the degradation of the constituent cell walls of blood vessels and xylem parenchyma, the development of chlorotic and necrotic sites and reduces the production of photosynthetic assimilates. Bacterial stress suppresses the physiological activity of tomato plants, which causes an imbalance in their nutrition. As a result, excess nitrogen reduces the pH of cell juice as a source of nutrition for pathogens. Deficiency of К+ and Са2+ leads to a decrease in the turgor potential and the formation of thin cell walls, through which phytopathogens easily penetrate, and the lack of Zn2+, Mn2+, Cu2+ and Fe2+ disrupts the accumulation of protective products of plant secondary compounds, in particular, phytoalexins and flavonoids [2]. Plants respond to infection with phytopathogens by a whole cascade of protective reactions such as the formation of barriers to the pathogen penetration by means of ligneous dead tissues, the closure of stomata in the light [5]. Under these conditions, plants change their gene expression and protein phosphorylation/ dephosphorylation patterns, activate ionic fluxes as well as the biosynthesis of protective substances and activate the synthesis of signaling molecules such as salicylic and jasmonic acids, ethylene and ROS. Overall, these changes lead to the strengthening of the cell wall and the accumulation of phytoalexins and Pathogen-dependent proteins (PR) [6, 7].
In recent years, the world's scientific centers effectively carry out biocontrol and protection of cultivated plants from bacterial pathogens by exogenous treatment of seeds and seedlings with biopreparations based on PGPB, which, thanks to the active colonization of roots, can directly or indirectly optimize plant performance and yield. These bacteria directly activate the processes of plant development as a result of fixation of atmospheric nitrogen, solubilization of iron and phosphorus, biosynthesis of siderophores, enzymes, phytohormones, in particular, indoleacetic acid, cytokinins and gibberellins [8, 9].
Indirectly, PGPB stimulate plant defence mechanisms against pathogenic microorganisms by inducing the synthesis of metabolites with antibiotic activity and of hydrolytic enzymes and by triggering mechanisms of systemic induced resistance through the synthesis of phytohormones [10-12]. It is in fact shown that PGPB can activate the mechanisms of systemic induced resistance at different levels of the plant organism [12].
However, the effects of PGPB in promoting tomato resistance towards bacterial pathogens remain poorly investigated.
The aim of our work was to determine and compare the morphological, anatomical and physiological-biochemical parameters of healthy tomato leaves, variety Chaika, with those of C. michiganensis subsp. michiganensis infected leaves both in the presence and absence of PGPB (B. subtilis, A. chroococcum or P. fluorescens) pretreatment.
2. MATERIALS AND METHODS
B. subtilis, P. fluorescens and A. chroococcum were obtained from the collection of microorganisms of the Department of Phytopathogenic Bacteria of the Zabolotny Institute of Microbiology and Virology, Natl. Academy of Sciences of Ukraine. Cell suspensions from these microorganisms were produced as described [13]. The ІZ-38 strain of C. michiganensis subsp. michiganensis isolated in Kyiv region [14] was used to infect the plants.
Small-scale field experiments were carried out in 4-fold repetition at the experimental field “Fruit and Vegetable Garden” of the National University of Life and Environmental Sciences of Ukraine. Soil characteristics were as follows: typical light-clay black soil, humus content in the topsoil 4.6%, pH 5.6. The object of the study was the seedlings of the Ukrainian variety Chaika which is characterized by short growing, early-ripening, high productivity, resistance against fusarium and growth in the open field. In this work, five groups of plants were tested: 1 – plants without treatments (healthy control), 2 – plants infected with C. michiganensis subsp. michiganensis ІZ-38 (infected control), 3 – plants treated with a suspension of B. subtilis cells (109 CFU/cm3) and infected with C. michiganensis subsp. michiganensis ІZ-38, 4 – plants treated with a suspension of P. fluorescens cells (109 CFU/ml3) and infected with C. michiganensis subsp. michiganensis ІZ-38, 5 – plants treated with the suspension of A. chroococcum (109 CFU/cm 3) and infected with C. michiganensis subsp. michiganensis ІZ-38. Tomato plants in the 3-4 leaf phase were treated with a cell suspension of PGPB (B. subtilis, A. chroococcum or P. fluorescens) [13] by spraying the plants in the morning, the rate of suspension was 200 ml per 1m2. The size of the registration area was 20 m2, the spatial isolation of the plots was ensured by sowing grain crops in strips 2 m wide.
Artificial infection of tomato stems and leaves was carried out using a suspension of C. michiganensis bacteria with a density of 1х109 CFU per ml of sterile tap water [13], after 24-48 hours pretreatment of PGPB (B. subtilis, A. chroococcum or P. fluorescens).
The level of disease development was assessed according to the five-point scale: 1 class – individual spots on the leaves at the injection site; 2 class – 1/4 of the surface of the infected leaves is covered with lesions; 3 class – spots can be counted, lesions cover not more than 1/3 of the surface of the leaves and stems; 4 class – lesions cover 2/3 of the surface of the leaves and stems; 5 class – wilting of leaf tops of the plants, stems of plants are greatly affected. The Disease progression Index (DI) was calculated according to the formula: DI= (1n1 + 2n2 + 3n3 + 4n4 + 5n5) 100 / 5nt, where n1-n5 is the number of plants in classes and nt is the total number of tested plants. The percentage of protective effect was calculated by the formula: resistance, % = A-B/A × 100%, where A = DI in the infected control, B = DI in the treated plants. For morphological, histological and biochemical analyses leaves were sampled at different height after 32 and 47 days of cultivation [15].
Anatomical and histological studies of bacterial pathogenesis of tissues were carried out according to the standard protocols [16]. The plant material was fixed with Chamberlain solution (70% ethanol–formalin–acetic acid, v/v/v – 90/5/5). The samples were dehydrated in a series of alcohols, which were replaced with chloroform and impregnated with paraffin. The experiments were performed on permanent micro-preparations with a thickness of 10-12 µm, which were made on a sledge microtome. Tissues were stained with aceto-basic fuchsin and hematoxylin according to Heidenhain. The linear dimensions of tomato leaf tissues and cells were measured using a specialized computer program Image Pro Premier 9.1 (Media Cybernetics,USA).
The net productivity of photosynthesis (weight gain of dry matter per unit time and per unit area of the leaves), chlorophyll index (the product of leaf area of the plant and the content of their total chlorophyll) and the specific surface leaf density (ratio between dry mass and leaf area), all parameters important to test the efficiency of the photosynthetic process, were evaluated [17].
To study the pigment complex, a sample of 1g of the leaves at different height was taken and ground in 10 ml of acetone. The amount of chlorophyll a (at a wavelength of 662), chlorophyll b – (at 644 nm) and carotenoids (k) (at 440 and 644 nm) were measured with the help of scanning spectrophotometer Оptizen POP (South Korea) and were calculated using the formulas [17, 18].
Soluble polyphenols were estimated by Folin Ciocalteau method in the modification of Singleton Rossi [19]. Polyphenols in plant extracts react with the Folin-Ciocalteu reagent to form a blue complex that can be quantified by visible-light spectrophotometry. Plant material was extracted with 100% MeOH at a ratio of 1:10. The amount of phenol was calculated by the formula:
![]() |
where F is the total content of intracellular phenolic compounds, mg∙g-1; C – is the concentration of phenolic compounds, l; V – extraction is the total volume of extraction, ml; m – is the mass of a sample, g.
The flavonoids content was determined by spectrophotometric method, using quercetin as standard [16]. The analysis was performed in the presence of aluminum chloride and sodium acetate, which form stable complexes with flavonoids. Catechins were evaluated by the spectrophoto-metric method using 9N H2SO4 and 1% vanillin [16].
Antioxidant activity of plant phenols was established by a modified method of Blois and Brand-Williams in the presence of 2,2-diphenyl-1-picrylhydrazyl (DFPH) [17]. Antioxidant activity was calculated by the formula:
![]() |
where Dk is an optical density without antioxidants (control), Do is an optical density in the presence of antioxidants (for calibration curve – Trolox in certain concentrations).
Peroxidase enzyme activity in leaves of tomato plants was measured using spectrophotometric method according to the optical density of the reaction products formed by oxidation of benzidine per second for 120 s at wavelength 590 nm. Samples of 200-300 mg were ground in cold porcelain mortar with a cold pestle in 2 ml of acetate buffer (pH 5.0). The resulting homogenates were centrifuged for 5 minutes at 12 000 g, and supernatants stored in a refrigerator at 4° C before the analysis. The peroxidase reaction mixture contained 150 ml of 0.2 M Na-acetate buffer (pH 5.0), 150 ml of 0.01% solution of muriatic benzidine, 50 ml of plant extract, 200 ml of 0.3% hydrogen peroxide and 200 ml of distilled water. The control cuvette contained 150 ml of 0.2 M Na-acetate buffer (pH 5.0), 150 ml of 0.01% solution of muriatic benzidine, 50 ml of extract and 400 mcl of distilled water. The rate of blue color development is a direct measure of the rate of the peroxidase reaction and the increase in absorbance is directly proportional to the peroxidase activity.
The peroxidase enzyme activity was calculated as follows:
![]() |
where E is an extinction = 0.125; a – the ratio of liquid taken for extract cooking, cm3/mg; B – the degree of constant dilution of extract in the reaction mixture; c – layer thickness, cm; t – time, s [17].
Statistical analyses were performed using the STATISTICA v. 6.0 application software package. The significance (р ≤ 0.05) of the data was calculated using the Student t-test.
3. RESULTS AND DISCUSSIONS
C. michiganensis subsp. michiganensis ІZ-38 induced various types of damages, in particular chloroses (mottled, interveinal, marginal), wrinkling and twisting, necroses of individual sections or of the entire surface of the leaves (Fig. 1). The nature of destructive disorders in the tissues of the infected tomato leaves varied based on the severity of the disease. Thus, at the initial stages of the disease, there was a vague oppression of the growth of young leaves. At the period when the obvious signs of bacterial disease were observed, there was a profound transformation of the epithelial tissues of the leaves, particularly of the palisade mesophyll.
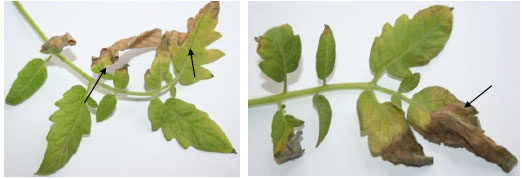
As a result of the bacteria damage, the cells of the tomato leaf mesophyll were deformed, the cytoplasm was less dense, and the chloroplasts on the leaves were reduced in size. The content of palisade and spongy parenchyma gradually collapsed with the formation of local chlorotic sites (Fig. 2).
The tomato leaf areas significantly affected by the bacterial canker pathogen firstly showed the deposition of waxy substances on the outer anticlinal cell walls and then the thickening and lignification of walls. As a result, structural and physiological isolation of healthy leaf tissues from damaged areas was created. However, in conditions of insufficient immune mobilization and low resistance of tomato plants against the pathogen, the degradation of the assimilation apparatus began, which predetermined the mass destruction of mesophyll cells (Table 1). Thus, external manifestations of chlorosis and necrosis of the leaf blade appeared. In particular, infected tomato leaves showed destroyed mesophyll, parenchyma, epidermal cells and vascular bundles. The upper and lower leaf epidermis was significantly compacted with smaller cells. The cells of the lower epidermis were characterized by growth abnormalities when compared to healthy ones (Fig. 2).
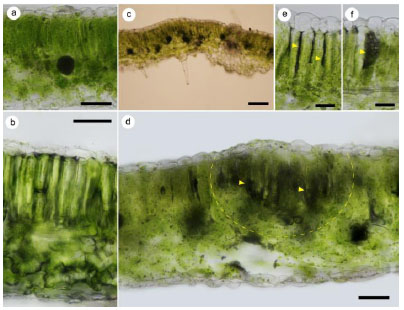
Indicators | Healthy Plants | Infected Plants | PGPB Treated Plants Infected with C. Michiganensis | ||
---|---|---|---|---|---|
Bacillus Subtilis | Pseudomonas Fluorescens | Azotobacter Chroococcum | |||
Leaf blade thickness | 158.1±1.42 | 148.1±1.67¤ | 170.2±1.12*¥ | 163.4±1.22*¥ | 165.2±1.33*¥ |
Thickness of columnar mesophyll | 61.2±0.56 | 58.1±0.19¤ | 67.3±0.82*¥ | 64.0±0.91*¥ | 65.2±0.84*¥ |
Thickness of spongy mesophyll | 78.5±0.42 | 58.7±0.74¤ | 84.5±0.12*¥ | 80.1±0.21*¥ | 82.3±0.36*¥ |
Ratio of thickness of columnar and spongy mesophyll | 0.78±0.04 | 1.09±0.06¤ | 0.80±0.02* | 0.79±0.02* | 0.79±0.03* |
Height of columnar mesophyll cells | 60.2±1.0 | 58.8±0.84 | 64.8±0.8*¥ | 62.3±0.6*¥ | 64.4±0.6*¥ |
Width of columnar mesophyll cells | 7.1±0.16 | 12.4±0.21¤ | 8.6±0.12*¥ | 8.2±0.10*¥ | 8.0±0.08*¥ |
Height of spongy mesophyll cells | 18.1±0.66 | 15.5±0.65¤ | 27.4±0.56*¥ | 23.6±0.60*¥ | 25.6±0.63*¥ |
Width of spongy mesophyll cells | 10.8±0.44 | 8.5±0.35¤ | 15.0±0.42*¥ | 13.8±0.40*¥ | 13.4±0.40*¥ |
Height of the cells of upper epidermis | 10.6±1.31 | 15.9±0.78¤ | 18.2±1.28¥ | 19.6±1.30¥ | 19.2±1.31¥ |
Height of the cells of lower epidermis | 7.1±0.30 | 9.8±0.31¤ | 10.4±0.18¥ | 10.6±0.20¥ | 10.8±0.16*¥ |
¤ ‒ statistically significant differences between the infected plants and the healthy plants.
Treatment of plants of the tomato variety Chaika with cell suspensions of PGPB B. subtilis, P. fluorescens or A. chroococcum reduced the development index of disease caused by C. michiganensis subsp. michiganensis ІZ-38 with respect to the C. michiganensis infected control plants, for which the percentage of damage was 67% (Table 2). The thickness of columnar and spongy mesophyll significantly decreased in C. michiganensis subsp michiganensis-infected plants with respect to the control, whereas the treatment with PGPR caused a significant increase in these values (Table 1). Likewise, the height of spongy and columnar mesophyll cells increased in C. michiganensis subsp michiganensis -infected plants treated with PGPR (foliar treatment). Many of the anatomical parameters considered significantly increased with PGR treatment. Also, in both C. michiganensis subsp michiganensis -infected and the infected plants in response to the pre-treatment with PGPB, the height of the cells of upper and lower epidermis increased significantly, with respect to healthy plants.
Treatment of Plants | Classes | DI, (%) |
Resistance (%) | ||||
---|---|---|---|---|---|---|---|
1 | 2 | 3 | 4 | 5 | |||
Infected control | 4 | 4 | 4 | 5 | 8 | 67 | 0 |
Bacillus subtilis | 10 | 7 | 5 | 2 | 1 | 42 | 38 |
Azotobacter chroococcum | 10 | 7 | 4 | 3 | 1 | 42 | 37 |
Pseudomonas fluorescens | 8 | 6 | 4 | 4 | 3 | 50 | 25 |
The use of cell suspensions of PGPB prevented complex structural changes. In particular, mesophill cells were cylindrical and densely packed in two layers, spongy parenchyma contained two or three cell layers. Also, under this condition, there was an increment in the number of protective biopolymers in epidermal cells and xylem and phloem tissues developed. Under control condition, the cuticle growth on the ab- and adaxial epidermal surfaces of tomato leaves with a smooth profile was not expressed, which is typical for mesophytes. On the basis of the conducted experiments, it was concluded that the thickening of cuticle is one of the most informative structural indicators of infected leaves. By thickening the cuticle, tomato plants ensure the constitutional stability against the causative agent of bacterial canker.
The resistance of plants against pathogenic bacteria is largely determined by the physiological state of the assimilation apparatus, and the levels of plastid pigments are markers of plant health. The total content of chlorophylls (a+b) in the infected C. michiganensis subsp. michiganensis ІZ-38 tomato leaves were significantly reduced, with respect to healthy plants. Conversely, the use of PGPB caused a statistically significant increment in the total amount of chlorophylls a+b when compared to the infected ones, regardless of the suspension cells used; this content ranged from 2.91 ± 0.05 (A. chroococcum) to 4.1±0.09 mg/g (B. subtilis). This increment likely ensures a high activity of the photosynthetic apparatus and the acquisition of the phenomenon of resistance towards bacterial canker (Table 3).
In response to the infection with C. michiganensis a significant decrease in the carotenoid content was measured, with respect to healthy plants. However, in the leaves of infected plants treated with cell suspensions of PGPB, the amount of carotenoids was significantly higher than in healthy and infected controls.
Then, the influence of PGPB on chlorophylline index was determined. B. subtilis, A. chroococcum and P. fluorescens treatments resulted in anincrement of chlorophilic index in the leaves by a factor of 1.36 - 1,43, and of the net productivity of photosynthesis by a factor of 1.74 – 1,87 with respect to C. michiganensis infected plants.
In the leaves of control plants, phenols were 22.74 mg/g (Table 4).
Phenols increased by 17.2% in plants infected with C. michiganensis and the use of PGPB further enhanced their levels (Table 4). The highest accumulation of phenols was found as the result of plant treatment with A. chroococcum. The level of catechins in the leaves of tomato control plants was 2.92 mg/g, it slightly increased following pathogen infection, to peak in plants treated with plant growth promoting bacteria (Table 4).
Indicators | Healthy Plants | Infected Plants | Treatment of Plants Before Infection |
||
---|---|---|---|---|---|
Pseudomonas Fluo-rescens |
Bacillus subtilis |
Azotobac-ter chro-ococcum | |||
Specific surface density of a leaf, mg/sm2 | 4.78± 0.18 |
4.05± 0.14¤ |
5.58± 0.24*¥ |
5.88± 0.22*¥ |
5.74± 0.24*¥ |
Total chlorophyll а+b, mg/g | 2.23± 0.05 |
1.88± 0.02¤ |
2.91± 0.05*¥ |
4.1± 0.09*¥ |
3.56± 0.08*¥ |
Carotenoids k, mg/g | 0.83± 0.03 |
0.65± 0.03¤ |
1.13± 0.04*¥ |
1.31± 0.08*¥ |
1.21± 0.03*¥ |
Total chlorophyll а+b/k | 2.69± 0.04 |
2.5± 0.03¤ |
3.06± 0.03*¥ |
3.13± 0.03*¥ |
3.21± 0.04*¥ |
Chlorophylline index, g/m2 | 1.31± 0.06 |
1.12± 0.04¤ |
1.52± 0.04*¥ |
1.58± 0.05*¥ |
1.6± 0.03*¥ |
Net productivity of photosynthesis, g/m2•day | 8.75± 0.32 |
7.6± 0.24¤ |
13.2± 0.42*¥ |
14.2± 0.36*¥ |
13.8± 0.34*¥ |
Treatment of Plants | Phenols, mg/g | Catechins, mg/g | Flavonoids, mg/g | Antioxidant Activity of Phenols, µm-EQ |
---|---|---|---|---|
Infected plants | 27.4±0.2¤ | 3.05±0.03¤ | 1.51±0.02¤ | 11.1±0.5¤ |
Healthy plants | 22.7±0.2 | 2.92±0.04 | 1.43±0.02 | 6.5±0.4 |
Bacillus subtilis | 41.0±0.6*¥ | 8.14±0.05*¥ | 1.68±0.03*¥ | 12.6±0.4¥ |
Azotobacter chroococcum | 54.1±0.6*¥ | 14.58±0.05*¥ | 2.06±0.02*¥ | 14.8±0.6*¥ |
Pseudomonas fluorescens | 33.9±0.5*¥ | 5.78±0.06*¥ | 1.61±0.03*¥ | 13.3±0.6*¥ |
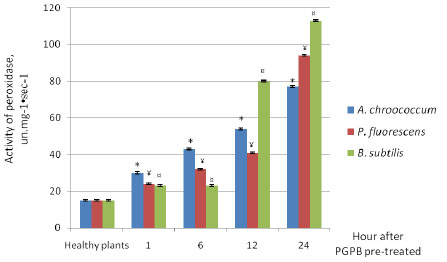
Quantitative and qualitative composition of flavonoids depends on species and varietal characteristics of plants, intensity and duration of environmental stress factors [20]. In healthy leaves, the total levels of flavonoids were 1.43 mg/g, whereas the infection caused an increment of these metabolites of 5.3%. Treatment with PGPB provided an additional increment of flavonoids, up 36% in comparison to the healthy controls (Table 4).
The effect of phytotoxic metabolites of bacterial pathogens on the structure of cell membranes is associated with the development of oxidative stress, which occurs due to the increased formation of ROS accompanied by damage to the structure and functions of polar membrane lipids of chloroplasts, nucleic acids and inactivation of enzyme systems [21, 22]. An antioxidant protection system plays a key role in the regulation of ROS in cells, which prevents oxidation of intracellular organic substances, the implementation of the protective action of biological structures and detoxification of secondary metabolites [21, 22].
The antioxidant activity of phenols in the tissues of tomato plants in a healthy control was 6.9 ± 0.4 µm-EQ, increased upon infection with C. michiganensis up to 11.1±0.5 µm-EQ and more consistently when plants were treated with PGPB (Table 4).
To study the adaptive properties of tomato plants, the dynamics of peroxidase enzyme activity was traced daily. In these experiments, it was found that the activity of peroxidase in leaves of plants without PGPB treatment remained at the initial level during the day – 15–15.6 un.mg-1•sec-1 (Fig. 3). As a result of treatment with PGPB, an increment in the activity of peroxidase was observed at 1,6, 12 and 24 h. In the leaves of plants treated with B. subtilis, activity of peroxidase reached the maximum value at 24 h (112.7 un.mg-1•sec-1). When A. chroococcum was used, the activity of peroxidase increased over the time up to 24 h (77.7 un.mg-1•sec-1). In our view, such an increase in peroxidase activity gives plants resistance to bacterial pathogens. In line with this, we observe that peroxidases are generally down regulated in Clavibacter michiganensis ssp. Michiganensis infected plants but their activity can be increased in Clavibacter michiganensis infected plants treated with chemicals that enhance the plant’s own defenses towards this pathogen [34].
4. DISCUSSION
The resistance of plants against pathogens of bacterial diseases can be increased by microbial products based on living cells and/or their fermentation products as an active ingredient [23]. The present study shows that the use of the PGPB B. subtilis, A. chroococcum or P. fluorescens, reduces the index of plant disease development on tomato leaves infected with C. michiganensis subsp. michiganensis. Similar results were obtained by other authors [24], who reported that PGPB produces biologically active antibacterial metabolites (antibiotics, toxins, surfactants -biosurfactants- and enzymes) to suppress the development of phytopathogens on tomato plants.
Our observations show that the upper and lower epidermis of the C. michiganensis infected tomato leaves is formed by small, especially dense cells, with the lower cells of the epidermis showing hyperplasia and hypertrophy with respect to cells of healthy leaves. We also show for the first time that, upon infection with C. michiganensis, abnormal spongy mesophyll is formed, while are absent cells of columnar parenchyma and chlorenchyma in the tomato leaves. Under this condition, mesophillic cells are relatively small and without intercellular spaces. Conversely, plant inoculation with PGPB triggers the accumulation of biopolymers with protective functions in epidermal cells, which is consistent with the phenomenon of activation of HR-specific genes published in the literature [23]. At the same time, PGPB induces an active growth of xylem and phloem tissues, which provide effective protection against the spreading of the pathogen and an impenetrable zone for the free transportation of nutrients for the pathogen. There is evidence [23] that the thickness, strength and elasticity of the outer wall of epidermal cells are important factors for tomato plant resistance against individual pathogens.
As a result of the infection with C. michiganensis, the cells of the leaves of the tomato variety Chaika, show significant degradation of chlorophyll when compared to the healthy ones. According to a few studies [25, 26], reactive oxygen species are formed during the pathogen lesion, which cause significant damage to chlorophyll and reduce the efficiency or halt the photosynthetic processes. The carotenoid content also decreases in the infected tomato leaves. Conversely, the treatment with the PGPB stimulates the accumulation of both chlorophyll a+b and carotenoids. Likely, this is a marker of the occurrence of a systemic resistance mechanism triggered by PGPB and of resistance of tomato to the infection of the pathogen.
Phenolic compounds play an important role in the regulation of metabolic processes and lignin biosynthesis [27], acting as free radical acceptors and substrates for antioxidant enzymes [28, 29]. In leaves of plants infected with C. michiganensis their levels increasemore in plants treated with PGPB. Phenolic compounds are involved in the accumulation of phytoalexins and the formation of structural barriers and mechanisms of plant resistance against pathogens [30-32]. In this regard, the metabolism of phenols and the lignification of the cell wall appear to be essential elements in plant protection against pathogenic bacteria [32].
A determining role in system-induced plant resistance is played by enzymes such as peroxidase, polyphenoloxidase, superoxide dismutase and proteinase inhibitors that induce initial reactions for the biosynthesis of phytoalexins and phenolic compounds [31]. In our experiments, the activity of peroxidase significantly increased in the leaves of tomato plants treated with PGPB. In particular, the activity of peroxidase was higher when leaves were treated with B. subtilis. Likely, the increased activity of antioxidant enzymes against pathogenic bacteria occurs with the help of a system of transduction of signal molecules such as jasmonic and salicylic acids to induce the biosynthesis of protective substances such as polyphenols, alkaloids and pathogenesis PR-proteins [30, 31, 33].
CONCLUSION
The use of cell suspensions of PGPB increased the resistance of tomato plants of the variety Chaika against the causative agent of bacterial canker. In particular, the treatment with PGPB induced an increment of the activity of antioxidant system components in C. michiganensis uninfected tomato plants and an increment of the content of chlorophylls and carotenoids, as well as of the activity of phenolic compounds in C. michiganensis infected tomato plants.
ETHICS APPROVAL AND CONSENT TO PARTICIPATE
Not applicable.
HUMAN AND ANIMAL RIGHTS
No animals/humans were used for studies that are the basis of this research.
CONSENT FOR PUBLICATION
Not applicable.
AVAILABILITY OF DATA AND MATERIALS
Not applicable.
FUNDING
None.
CONFLICTS OF INTEREST
The authors declare no conflict of interest, financial or otherwise.
ACKNOWLEDGEMENTS
Declared none.