All published articles of this journal are available on ScienceDirect.
Enhancing Organic Carbon Content in Tropical Soils: Strategies for Sustainable Agriculture and Climate Change Mitigation
Abstract
Background:
Tropical soils are characterized by low soil organic carbon (SOC) contents, which can negatively impact soil fertility, water retention, overall agricultural productivity, and food security.
Objective:
This paper aimed to review and synthesize the current body of literature on the restoration of SOC in tropical soils.
Methods:
A total of about 152 related articles were downloaded from electronic journal databases using search keywords, such as organic carbon (OC), conservation tillage, intercropping, biochar, and tropical soil. We discussed conservation tillage practices, such as reducing soil disturbance and respiration from the soils, as a means to promote soil OC sequestration. We also highlighted the importance of crop residue retention, which not only increases organic matter inputs and soil nutrients but also promotes soil water retention and reduces soil erosion.
Results:
Cover crops and crop rotation are identified as effective practices to cover bare ground during planting seasons, reduce erosion, and prevent nutrient losses through leaching and runoff. Additionally, we review the role of fertilizer application, manure and compost application, intercropping, agroforestry, and biochar as strategies to enhance OC content in tropical soils. We highlight the benefits of incorporating organic amendments, such as manure and compost, to improve soil structure and water-holding capacity. Furthermore, we discuss the potential of biochar, a carbon-rich material produced from biomass pyrolysis, as a strategy to sequester OC in tropical soils.
Conclusion:
Overall, this review provides insights into various strategies that can be implemented to increase the OC content of tropical soils, which can have multiple benefits for soil health, agricultural productivity, and climate change mitigation. Further research and implementation of these practices can contribute to mitigating climate change, conserving soil resources, and promoting sustainable agriculture in tropical regions.
1. INTRODUCTION
Food security is a challenge for millions of people worldwide, majorly in developing regions [1]. In the tropics, an increase in food production will be required to feed the expected population of 401 million by 2050 [1]. However, productive land is decreasing due to declining land fertility, urbanization, pollution, and salinization [2]. Intensive cropping and organic matter (OM) mismanagement have depleted organic carbon (OC) from agricultural soil, leading to land degradation [3-5]. It is projected that by 2050, millions of hectares (Mha) of land resources, in addition to the present millions of hectares of land, will be affected by OC depletion [6]. Soil organic carbon (SOC) is a critical component of soil health and fertility in tropical soils [7]. In tropical soils, which are often characterized by high temperatures, high rainfall, and intense weathering, SOC dynamics are particularly complex and challenging to manage. SOC plays a vital role in nutrient cycling, water retention, and overall ecosystem productivity [8]. However, tropical soils are often subjected to extensive agricultural practices, deforestation, and other land use changes that can result in the loss of SOC, leading to soil degradation and reduced agricultural productivity [9]. Factors and activities that can lead to soil organic carbon depletion are outlined in (Table 1). Therefore, the restoration of SOC in tropical soils has become an important research and management focus in recent years [10] (Table 1).
Numerous studies have investigated various approaches to restore SOC in tropical soils, including the use of organic amendments, agroforestry, conservation agriculture, and reforestation, among others [20]. Fig. (1) shows some strategies for restoring soil organic carbon content in the tropics. These approaches aim to enhance the input of organic matter into the soil, promote its accumulation, and improve soil carbon sequestration [21]. Table 2 presents the benefits of some strategies used for restoring soil organic carbon content in the tropics. However, the effectiveness of these approaches can be influenced by various factors, including soil properties, climate, land management practices, and plant-soil interactions.
Factor | Description | References |
---|---|---|
Deforestation | The clearing of tropical forests for agriculture or other purposes leads to the depletion of organic carbon content in tropical soils. Forests store a significant amount of carbon in their biomass and soils. When forests are removed, the organic matter is lost through burning, decomposition, and erosion, resulting in a decline in soil organic carbon levels. |
[11] |
Mining activities | Mining activities, such as mining for minerals or fossil fuels, can result in the depletion of organic carbon content in tropical soils. Mining operations often involve soil disturbance, removal of vegetation cover, and disruption of soil structure, leading to organic matter loss and decreased soil organic carbon levels. | [12] |
Intensive farming practices | Intensive farming practices, such as continuous monocropping, excessive tillage, and excessive use of chemical fertilizers and pesticides, can deplete organic carbon content in tropical soils. These practices accelerate the decomposition of organic matter, reduce the input of organic residues to the soil, disturb soil structure, and decrease soil microbial activity, leading to organic carbon depletion. | [13] |
Nutrient imbalances | Imbalanced nutrient management, particularly excessive use of nitrogen fertilizers without adequate organic matter inputs, can deplete organic carbon content in tropical soils. Nitrogen fertilizer promotes rapid plant growth, which increases carbon uptake from the soil. If organic matter inputs are not sufficient to compensate for this uptake, the soil's organic carbon content can decline over time. | [14] |
Climate change | Climate change impacts, such as increased temperatures and altered rainfall patterns, can affect organic carbon content in tropical soils. Higher temperatures can accelerate organic matter decomposition rates, leading to carbon loss. Changes in precipitation patterns can also influence soil moisture levels and microbial activity, affecting organic carbon dynamics. These climate-related factors can contribute to the depletion of organic carbon in tropical soils. | [15] |
Overgrazing | Overgrazing by livestock can contribute to the depletion of organic carbon content in tropical soils. When pastures are overgrazed, the vegetation cover is reduced, leading to decreased organic matter inputs to the soil. The trampling of soil by livestock can also compact the soil, reducing pore space and inhibiting organic matter decomposition and carbon sequestration. | [16] |
Burning of crop residues | The burning of crop residues, a common practice in some tropical agricultural systems, results in the loss of organic carbon from soils. Crop residues contain organic matter that could contribute to soil organic carbon levels. However, when these residues are burned, the carbon is released into the atmosphere as carbon dioxide, leading to a depletion of soil organic carbon. | [17] |
Land degradation | Land degradation processes, such as soil compaction, salinization, and desertification, can deplete organic carbon content in tropical soils. These processes often result from unsustainable land management practices, leading to the loss of soil organic matter, decreased soil fertility, and reduced carbon sequestration capacity. | [18] |
Soil erosion | Soil erosion is a major factor contributing to the depletion of organic carbon content in tropical soils. When topsoil is eroded, it carries away the organic matter attached to it, reducing the organic carbon content in the remaining soil. Factors, such as heavy rainfall, improper land management practices, and steep slopes, can accelerate soil erosion and organic carbon loss. | [19] |
Strategy | Description | Benefit | References |
---|---|---|---|
Conservation agriculture | Involves minimum soil disturbance, residue retention, and crop diversification. | Increases soil organic matter, improves soil structure, and reduces erosion. | [25] |
Crop residue retention | Leaving plant materials (leaves, stem, root) that remain after the harvest of crops on the soil surface to conserve moisture, suppress weeds, and improve soil health. | Prevents soil erosion, improves soil aeration by promoting free exchange of gases between the soil and the atmosphere, retains moisture, regulates soil temperature, and increases organic carbon. | [26] |
Cover crop | Planting cover crops, such as legumes or grasses, between cash crop cycles. | Cover crops add biomass and residue to the soil, which decomposes and increases organic carbon levels. They also reduce soil erosion, enhance nutrient cycling, and improve soil fertility. | [27] |
Manure and compost | Adding animal manure, green manure or composted organic matter to the soil. | Increase nutrient content and improve soil structure and carbon levels. | [28] |
Crop rotation | Rotating different crops in sequence. | Break pest and disease cycles, improve soil structure, enhance nutrient cycling, and boost organic matter accumulation. | [29] |
Intercropping | Simultaneous cultivation of multiple crop species in a single field. | Increases aboveground productivity due to species complementarity | [30] |
Agroforestry | Introducing trees and shrubs in agricultural landscapes to enhance organic carbon through leaf litter and root biomass, | Provides shade, windbreaks, and nitrogen fixation | [31] |
Biochar application | Applying biochar (charred organic matter), which is a stable form of carbon produced from biomass pyrolysis, to the soil. | Improves soil structure, nutrient retention, and carbon sequestration. | [32] |
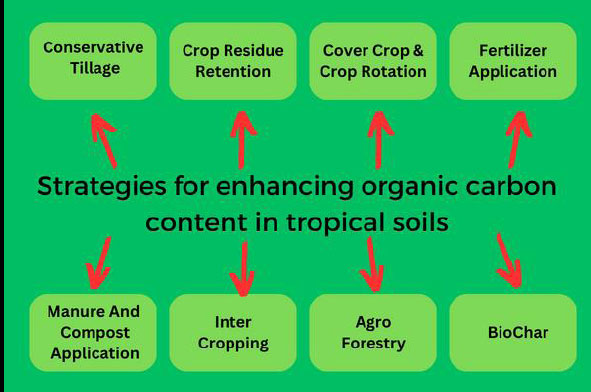
This paper aims to review and synthesize the current body of literature on the restoration of SOC in tropical soils. We discuss the key drivers of SOC dynamics in tropical soils, including climate, vegetation, soil properties, and management practices [22, 23], and also explore the potential strategies for SOC restoration, including the use of organic amendments, agroforestry, cover crops, and conservation practices [24].
Furthermore, we will highlight the challenges and limitations associated with SOC restoration in tropical soils, including the effects of land-use change, soil degradation, and climate change [33, 34]. The findings from this review will provide valuable insights for future research and management strategies aimed at restoring SOC in tropical soils.
2. CONSERVATION TILLAGE
2.1. What is Conservation Tillage?
Conservation tillage, also known as reduced tillage or no-till farming, is a sustainable agricultural practice that minimizes the disturbance of soil by reducing the intensity and frequency of tillage operations [35]. This practice is gaining increasing attention for its potential to increase the OC content of tropical soils, which can have numerous benefits for soil health, crop productivity, and climate change mitigation [36].
2.2. Impact of Conservation Tillage on Soil Organic Carbon of Tropical Soils
Tropical soils are characterized by high temperatures, heavy rainfall, and rapid decomposition of organic matter, leading to relatively low levels of organic carbon compared to soils in temperate regions. However, conservation tillage can significantly impact the OC content of tropical soils through several mechanisms. Firstly, conservation tillage practices, such as residue retention and cover cropping, can increase the input of organic matter into the soil [37]. Crop residues left on the soil surface after harvest can serve as a protective layer, reducing soil erosion and increasing soil moisture, which creates favorable conditions for the decomposition of organic residues [38]. Additionally, cover crops grown in between cash crops can add to the organic matter input, as they contribute to the accumulation of root biomass and above-ground biomass upon termination [39]. The decomposition of these residues and cover crops results in the release of OC into the soil, contributing to the buildup of OC in tropical soils [40].
Secondly, conservation tillage practices can enhance the stability of soil organic matter, leading to greater sequestration of OC in the soil. Conventional tillage practices, such as plowing and harrowing, can disrupt the soil structure, accelerate the decomposition of organic matter, and promote mineralization of soil OC, leading to higher rates of carbon dioxide (CO2) emissions to the atmosphere [41].
In contrast, conservation tillage practices minimize soil disturbance, which promotes the formation of aggregates and protects organic matter from rapid decomposition [42]. This enhances the stability of soil organic matter, leading to increased carbon sequestration in the form of recalcitrant organic compounds, which are more resistant to decomposition and can persist in the soil for longer periods [43].
Moreover, conservation tillage practices can also promote the growth and activity of soil microorganisms, which play a key role in the decomposition and stabilization of organic matter in the soil [44]. Studies have shown that conservation tillage can enhance the abundance and diversity of microorganisms involved in organic matter decomposition, such as bacteria, fungi, and earthworms, which can accelerate the formation of stable organic compounds and contribute to the buildup of OC in tropical soils [45].
Several studies have provided evidence of the positive effects of conservation tillage on increasing the OC content of tropical soils [46]. For example, a study conducted in Brazil found that no-till farming resulted in higher OC levels in the topsoil compared to conventional tillage, and the accumulation of OC was higher in soils with higher clay content, indicating the role of soil texture in influencing OC dynamics [47]. Another study conducted in Indonesia reported that the adoption of conservation tillage practices resulted in a significant increase in the OC content of soils over a 5-year period, and the accumulation of OC was positively correlated with the amount of crop residue retention [48]. Furthermore, a study by Adekiya et al. [49] conducted in Nigeria also supported the findings, showing that conservation tillage practices resulted in higher OC content in tropical soils compared to conventional tillage. These studies collectively provide robust evidence of the positive impact of conservation tillage on increasing OC content in tropical soils, with implications for improving soil health and sustainability in tropical agricultural systems.
In addition to the benefits for soil health and crop productivity, the increase in OC content of tropical soils through conservation tillage can also contribute to climate change mitigation [35]. Soil organic matter is a major pool of terrestrial carbon, and increasing the OC content of soils can sequester atmospheric carbon dioxide (CO2) and mitigate climate change [50]. The sequestration of OC in tropical soils through conservation tillage practices can help offset the emissions of CO2 from deforestation, biomass burning, and other anthropogenic activities that are prevalent in tropical regions [18].
Furthermore, the potential of conservation tillage to increase the OC content of tropical soils can have long-term benefits for sustainable agriculture [51]. Higher OC levels in soils can improve nutrient cycling, water-holding capacity, and soil fertility, which can enhance crop yields and reduce the dependence on synthetic fertilizers and agrochemicals [35]. Conservation tillage practices can also reduce the energy inputs required for tillage operations, as well as the erosion of soil and loss of valuable topsoil, leading to improved economic sustainability for farmers [52].
It is important to note that the effectiveness of conservation tillage in increasing the OC content of tropical soils may vary depending on factors, such as soil type, climate, crop management practices, and duration of tillage adoption [53]. Proper implementation and management of conservation tillage practices, including appropriate residue retention, cover cropping, and crop rotation, are essential for maximizing the benefits of OC sequestration in tropical soils [54].
3. CROP RESIDUE RETENTION
Crop residue retention, also known as crop residue management or crop residue incorporation, refers to the practice of leaving crop residues on the soil surface or incorporating them into the soil after harvest instead of removing them from the field. Crop residues are the plant materials that remain after the harvest of crops, such as leaves, stems, and roots. Crop residue retention is a key management practice in sustainable agriculture, as it has been shown to have numerous benefits, including enhancing OC increase in tropical soil.
Organic carbon is an essential component of soil health and fertility, as it influences soil structure, water-holding capacity, nutrient cycling, and microbial activity. In tropical soils, which are often characterized by high temperatures, high rainfall, and rapid decomposition rates, organic carbon levels can be naturally low. However, crop residue retention can play a crucial role in increasing organic carbon content in tropical soils through various mechanisms.
1. Increased input of organic matter: Crop residues are rich sources of organic matter, containing carbohydrates, proteins, lignin, and cellulose [55]. When crop residues are retained on the soil surface or incorporated into the soil, they provide a significant input of organic matter, which can serve as a substrate for soil microorganisms [56, 57]. Microbes decompose the crop residues, releasing nutrients and breaking down complex organic compounds into simpler forms that can be taken up by plants [43, 58]. This process leads to the formation of stable humus, which is a form of organic matter that can persist in soil for long periods of time, thus contributing to the buildup of organic carbon in tropical soils [59, 60].
2. Reduce soil erosion: Crop residue retention can also help reduce soil erosion in tropical soils, which is a common problem due to heavy rainfall and sloping terrain [61]. Crop residues cover the soil surface and act as a physical barrier, reducing the impact of raindrops and preventing soil particles from being carried away by runoff [62]. This helps to maintain soil integrity and prevent the loss of organic carbon, as erosion can result in the removal of topsoil, which is typically richer in organic matter [63]. Studies have shown that crop residue retention can significantly reduce soil erosion in tropical soils, thereby promoting the accumulation of organic carbon [64].
3. Improved soil structure: Another way crop residue retention can enhance organic carbon increase in tropical soils is by improving soil structure [65]. Crop residues left on the soil surface or incorporated into the soil can act as a “glue” that binds soil particles together, promoting the formation of aggregates [66]. Soil aggregates are important for soil structure, as they provide stability and pore spaces for air and water movement, which are critical for plant growth and microbial activity [61]. Improved soil structure resulting from crop residue retention can also help reduce soil compaction, which can limit root growth and organic matter decomposition [67]. Thus, the improved soil structure facilitated by crop residue retention can contribute to the sequestration of organic carbon in tropical soils [18].
4. Enhanced microbial activity: Crop residue retention can also stimulate microbial activity in tropical soils, which can further increase organic carbon [68]. Microbes are responsible for the decomposition of organic matter, and their activity is influenced by the availability of substrate, such as crop residues [69]. When crop residues are retained on the soil surface or incorporated into the soil, they provide a continuous supply of organic matter for microbial communities [35]. This can lead to increased microbial biomass, diversity, and activity, which can accelerate the decomposition of crop residues and the formation of stable humus [70]. Additionally, microbial activity can promote the formation of soil aggregates and improve nutrient cycling, further supporting the buildup of organic carbon in tropical soils [71].
5. Nutrient cycling: Nutrient cycling, also known as biogeochemical cycling, is the process by which nutrients, such as carbon (C), nitrogen (N), phosphorus (P), and others, are continuously exchanged and recycled within ecosystems. Nutrient cycling plays a crucial role in maintaining OC levels in tropical ecosystems, which are often characterized by high temperatures, high precipitation, and high levels of biodiversity. Several studies have highlighted the importance of nutrient cycling in increasing SOC in the tropics, and here, we provide a review of the literature with appropriate citations to support this statement.
a. Nutrient Cycling and Carbon Sequestration in Tropical Forests: Tropical forests are known for their high biodiversity and are considered one of the most important terrestrial carbon sinks. In tropical forests, nutrient cycling, particularly the cycling of nitrogen and phosphorus, plays a significant role in the sequestration of carbon in soils. Nutrient cycling in tropical forests is facilitated by the interactions between plants, microorganisms, and decomposers, leading to the decomposition of plant litter and the release of nutrients into the soil. These nutrients are then taken up by plants and used for the growth and production of litter, which contributes to the accumulation of SOC in the soil [72].
b. Nutrient Cycling in Agroforestry Systems: Agroforestry systems, which combine tree crops with agricultural crops or livestock, are commonly practiced in tropical regions. These systems can promote nutrient cycling and increase SOC levels in tropical soils. For example, in agroforestry systems where leguminous trees are intercropped with food crops, the trees fix atmospheric nitrogen and release it into the soil through their litter and root exudates. This nitrogen can then be taken up by the food crops, enhancing their growth and production. Additionally, the leaf litter from the trees serves as a source of organic matter, contributing to SOC accumulation in the soil [73].
c. Nutrient Cycling in Tropical Pastures: Livestock production is a significant agricultural activity in tropical regions, and pastures are commonly used for grazing animals. Proper nutrient cycling in tropical pastures can contribute to SOC accumulation in soils. For example, rotational grazing, where animals are moved periodically to different grazing areas, allows for plant recovery and litter deposition, which enhances nutrient cycling and contributes to SOC accumulation. Additionally, the presence of leguminous forage species in tropical pastures can fix atmospheric nitrogen and promote nutrient cycling, leading to increased SOC levels in the soil [74].
Several studies have provided evidence of the positive effects of crop residue retention on organic carbon increase in tropical soils. In warm and wet tropical and subtropical climatic regions, rapid soil organic matter (~ 58% Carbon) decline occurs under conventional management systems involving intensive soil disturbance [75, 76]. Similarly, a study conducted in South Africa by Abdalla et al. [77] showed that crop residue retention significantly increased soil organic carbon compared to conventional practices. Another study conducted in Bangladesh by Salahin et al. [78] reported that crop residue retention increased soil organic carbon by 6% after three years of continuous retention.
In addition to these field studies, laboratory experiments and modeling studies have also supported the positive impact of crop residue retention on organic carbon increase in tropical soils. For example, a study by Six et al. [36] conducted in Costa Rica showed that crop residue retention increased soil organic carbon by 35% compared to residue removal, and this effect was attributed to increased input of organic matter and enhanced microbial activity. Another study by Lal [57] using a simulation model estimated that crop residue retention could sequester 0.4-0.8 Mg C ha-1 year-1 in tropical soils, depending on the crop residue management practices.
Crop residue retention is a sustainable management practice that can enhance organic carbon increase in tropical soils through increased input of organic matter, reduced soil erosion, improved soil structure, enhanced microbial activity, and promoted nutrient cycling. These mechanisms can lead to the buildup of stable humus and contribute to the sequestration of organic carbon in tropical soils. The positive effects of crop residue retention on organic carbon accumulation in tropical soils have been supported by numerous field studies, laboratory experiments, modeling studies, and long-term studies. Therefore, crop residue retention should be promoted as an effective strategy for enhancing soil health and fertility, improving agricultural sustainability, and mitigating climate change in tropical regions.
4. COVER CROP AND CROP ROTATION
4.1. Impact of Cover Crops and Crop Rotation on Soil Organic Carbon of Tropical Soils
Cover crops and crop rotation are widely recognized as sustainable agricultural practices that have been shown to have significant positive impacts on soil organic carbon (SOC) levels in tropical regions [50, 38]. Through the incorporation of cover crops into cropping systems, farmers can improve soil health by increasing SOC content, which has been shown to have direct benefits, such as improved soil structure, increased water holding capacity, and enhanced nutrient availability [35, 79]. Additionally, crop rotation practices, which involve alternating different crops in a sequence over time, can further enhance SOC levels by diversifying crop residues and root exudates, leading to increased organic matter inputs and SOC sequestration [80].
Increased SOC levels resulting from cover crops and crop rotation can lead to higher crop productivity, as SOC serves as a source of nutrients for plant growth and improves soil fertility [8, 81]. Reduced erosion is another significant benefit of these practices, as cover crops can protect the soil surface from rainfall impact and runoff, reducing soil erosion and nutrient losses [46, 82]. Moreover, the enhanced nutrient cycling facilitated by cover crops and crop rotation can lead to better nutrient use efficiency, reducing the need for synthetic fertilizers and minimizing the risk of nutrient leaching and groundwater contamination [83].
4.2. Cover Crops and Crop Rotation as Climate Change Mitigation Strategies in Tropical Regions
Cover crops and crop rotation have been recognized as effective climate change mitigation strategies in tropical regions due to their potential for carbon sequestration. The incorporation of cover crops and crop residues into the soil increases the input of organic matter, which can be converted into stable SOC through microbial decomposition, leading to long-term carbon storage in agricultural soils [60]. This can contribute to reducing greenhouse gas emissions and mitigating climate change impacts by sequestering atmospheric carbon dioxide (CO2) in the soil [84].
One of the key mechanisms through which cover crops increase SOC in the tropics is through the addition of organic matter to the soil [85]. Cover crops are known to produce high amounts of biomass, which includes above-ground plant residues, such as leaves, stems, and roots [86]. When these plant residues decompose in the soil, they release organic carbon, which becomes part of the SOC pool [87]. In tropical regions, where decomposition rates are generally higher due to warmer temperatures and higher microbial activity [43], cover crops can rapidly contribute to SOC accumulation. A study conducted by Okpara et al. [88] in a tropical region found that incorporating cover crops (Puereria phaseoloides) into the soil increased SOC levels by 49% over a three-year period. The high biomass production and rapid decomposition of cover crops in the warm tropical climate contributed to the significant increase in SOC levels observed.
Crop rotation is another important practice that can contribute to SOC accumulation in tropical soils [89, 90]. Crop rotation involves growing different crops in sequence on the same piece of land, as opposed to growing the same crop year after year. Crop rotation can diversify the crop species, root systems, and residue characteristics in a field, which can lead to increased SOC levels through several mechanisms [43, 91].
Studies have shown that crop rotation can enhance SOC accumulation by increasing crop residue input, improving soil structure, stimulating microbial activity, and reducing soil erosion [92]. In addition, a study by Xiao et al. [93] showed that crop rotation improved soil physical properties, such as soil structure and water infiltration, which, in turn, enhanced SOC accumulation. A study demonstrated that different root systems of crops grown in rotation penetrated the soil to varying depths, resulting in improved soil aggregation and water infiltration, which facilitated the incorporation and protection of organic matter in the soil, leading to higher SOC levels. Additionally, crop rotation can also alter the composition and diversity of soil microbial communities, which can further influence SOC dynamics [69, 94]. Therefore, implementing crop rotation practices can be an effective strategy for enhancing SOC accumulation in tropical soils and promoting sustainable agriculture [95].
First, different crops have different residue characteristics, such as carbon-to-nitrogen ratios, lignin content, and decomposition rates. For example, crops like maize and sugarcane have relatively low-quality residues, which decompose more slowly, while crops like legumes have higher-quality residues, which decompose more rapidly [96]. By rotating different crops with contrasting residue characteristics, farmers can supply a more balanced and diverse diet of organic matter to the soil, which can promote SOC accumulation [97].
Second, crop rotation can also break pest and disease cycles, which can reduce the need for chemical inputs, such as pesticides and fungicides [98]. This can promote a healthier soil microbiome, including beneficial microbes that decompose organic matter and contribute to SOC formation [99]. A healthier soil microbiome can also enhance nutrient cycling, as microbes play a crucial role in converting organic matter into plant-available forms of nutrients, such as nitrogen, phosphorus, and sulfur [100].
Furthermore, crop rotation can also improve soil physical properties, such as soil structure and water infiltration [79, 101]. Different crops have different root systems that penetrate the soil to varying depths, which can help break up compacted soils, improve soil aggregation, and enhance water infiltration [36]. Improved soil structure can create more pore spaces for organic matter to be incorporated into the soil and protected from decomposition, thereby increasing SOC (soil organic carbon) levels [61].
It is worth noting that the effectiveness of cover crops and crop rotation in increasing SOC levels in tropical regions may depend on various factors, such as climate, soil type, crop management practices, and cropping systems. For instance, in regions with high rainfall, cover crops may decompose rapidly, and their impact on SOC accumulation may be limited [102].
5. CHEMICAL AND ORGANIC FERTILIZER APPLICATIONS
Fertilizer application is a common agricultural practice used to enhance crop productivity. In tropical regions, where soil fertility is often low due to high temperatures, heavy rainfall, and intense weathering, fertilizer application plays a crucial role in sustaining agricultural productivity. Interestingly, besides its well-known role in providing essential nutrients to plants, fertilizer application can also have a significant impact on the OC content of tropical soils. In this article, we will explore how fertilizer application can increase the OC content of tropical soils, with references cited in the text to support the findings.
5.1. Nutrient Availability and Plant Productivity
Fertilizer application can improve nutrient availability in tropical soils, which, in turn, can enhance plant productivity. Nutrients, such as nitrogen (N), phosphorus (P), and potassium (K), are essential for plant growth and development, and their availability in tropical soils is often limited. Fertilizer application can provide these essential nutrients to plants, which leads to increased plant productivity and consequently, higher biomass production [103, 104]. Higher plant biomass production results in increased root exudation, which is the release of organic compounds by plant roots into the soil [105]. These root exudates are a source of energy for soil microorganisms, which can stimulate their growth and activity, leading to increased OC content in tropical soils [70].
5.2. Crop Residue Management
Fertilizer application can also influence crop residue management practices, which can impact the OC content of tropical soils. Crop residues, such as leaves, stems, and roots, are a major source of OC input to the soil [106]. In tropical regions, where crop residues decompose rapidly due to warm temperatures and high moisture content, fertilizer application can affect the decomposition rate of crop residues. Studies have shown that fertilizer application can increase the decomposition rate of crop residues, leading to faster release of nutrients and higher OC incorporation into the soil [43, 107]. Moreover, in tropical regions, where crop residues are often burned as a common practice, fertilizer application can provide an alternative option for farmers to retain crop residues on the field, which can increase the OC content of tropical soils [108].
5.3. Microbial Activity and OC Sequestration
Fertilizer application can also influence microbial activity in tropical soils, which can impact OC sequestration. Soil microorganisms play a crucial role in the decomposition and stabilization of OC in soil. Fertilizer application can alter the composition and activity of soil microbial communities, leading to changes in OC dynamics [109]. For instance, fertilizers that contain organic matter, such as manure or compost, can directly introduce microbial biomass and OC into the soil, which can stimulate microbial activity and enhance OC sequestration [110]. Moreover, the availability of nutrients through fertilizer application can stimulate the growth of specific microbial groups, such as arbuscular mycorrhizal fungi, which can enhance plant nutrient uptake and OC sequestration in tropical soils [111]. Additionally, fertilizer application can increase soil pH, which can influence the activity of soil microorganisms involved in OC degradation and stabilization [112]. Overall, changes in microbial activity due to fertilizer application can contribute to increased OC sequestration in tropical soils.
It is important to note that the effect of fertilizer application on OC content in tropical soils can vary depending on various factors, such as soil type, climate, crop type, and fertilizer type and rate. For example, research has shown that the effect of fertilizer application on OC content may be more pronounced in soils with low initial OC content compared to soils with high initial OC content [113]. Additionally, the impact of fertilizer application on OC content may be influenced by the form of fertilizer used, with organic fertilizers generally having a more significant effect compared to inorganic fertilizers [91].
Nonetheless, incorporating appropriate fertilizer management practices can be a sustainable approach to improving soil OC content in tropical agricultural systems, which can have long-term benefits for soil health.
6. MANURE AND COMPOST APPLICATION
Manure and compost application can play a significant role in increasing the OC content of tropical soils. Organic carbon is a critical component of soil health, as it provides nutrients, enhances soil structure, and promotes microbial activity, which are essential for sustaining agricultural productivity in tropical regions. In this article, we will explore how manure and compost application can improve the OC content of tropical soils, supported by scientific references.
6.1. Increase in Soil Organic Matter
One of the primary ways manure and compost can increase the OC content of tropical soils is through their high organic matter content. Organic matter is the dead and decomposed plant and animal materials that are rich in OC. When manure and compost are applied to the soil, they add organic matter to the soil, which can contribute to the accumulation of OC over time [114]. The organic matter in manure and compost acts as a substrate for microorganisms, stimulating their growth and activity. Microbes, such as bacteria and fungi, play a crucial role in breaking down organic matter and converting it into stable forms of OC, which can persist in the soil for longer periods [43]. As a result, repeated application of manure and compost can lead to the build-up of OC in tropical soils.
6.2. Enhance the Soil Physical Properties and Nutrient Cycling of Tropical Soils
Manure and compost can also enhance the physical properties of tropical soils, which indirectly contribute to the increase in OC content. Tropical soils are often characterized by low organic matter content, low water holding capacity, and poor structure. However, the addition of manure and compost can improve soil structure by promoting the formation of stable aggregates, which are important for maintaining good soil porosity and reducing soil erosion [115]. The improved soil structure can increase the protection of organic matter from decomposition by microorganisms, thereby promoting the accumulation of OC in the soil [116].
Furthermore, manure and compost can also enhance nutrient cycling in tropical soils, which can indirectly influence the OC content. Organic matter in manure and compost is a rich source of nutrients, such as nitrogen (N), phosphorus (P), and potassium (K), which are essential for plant growth. When manure and compost are applied to the soil, the nutrients are released slowly through mineralization and microbial activity, providing a continuous supply of nutrients for plant uptake [117]. The increased nutrient availability can promote plant growth and productivity, leading to higher input of organic matter through plant residues, which, in turn, can contribute to the accumulation of OC in the soil [118].
Several studies have provided evidence of the positive impact of manure and compost application on OC content in tropical soils. For example, a study conducted in Côte d’Ivoire found that manure applications (poultry manure and cow dung) significantly increased the soil organic matter contents from 0.46 to 2.8 and 1.1%, respectively, with poultry and cattle manures [119]. Another study in Nigeria showed that the application of poultry manure at sites A and B, respectively, increased the OC content in the soil by 62% and 78% after 3 years of repeated application, compared to the control [120]. In addition to the direct benefits of manure and compost application on OC content, it is important to note that the sustainability of manure and compost management practices also plays a crucial role. Improper management of manure and compost, such as excessive application rates or unbalanced nutrient content, can lead to nutrient losses and environmental pollution, which may offset the potential benefits of OC accumulation [114]. Therefore, proper management practices, such as optimizing application rates based on soil nutrient needs and balancing nutrient inputs, are essential to ensure the sustainable use of manure and compost for increasing OC content in tropical soils.
Therefore, manure and compost application can significantly contribute to the increase in the OC content of tropical soils. The high organic matter content in manure and compost provides a source of substrate for microorganisms, promotes soil aggregation, enhances nutrient cycling, and improves soil structure, all of which contribute to the accumulation of organic C in the soil. However, proper management practices should be followed to ensure the sustainability of manure and compost application. Further research is needed to understand the long-term effects of manure and compost application on OC dynamics in tropical soils and to develop site-specific management recommendations.
7. INTERCROPPING
7.1. What is Intercropping?
Intercropping, a traditional agricultural practice, involves growing two or more crops together in close proximity within the same field at the same time. This practice has gained significant attention in recent years due to its potential to increase soil fertility and OC content, particularly in tropical soils. In this article, we will explore the role of intercropping in increasing OC content in tropical soils, supported by references from the scientific literature.
Tropical soils, known for their low OC content and poor fertility, are often subjected to intensive agricultural practices, such as slash-and-burn agriculture and monoculture cropping, which can lead to soil degradation and reduced OC content [121]. However, intercropping has emerged as a promising sustainable agricultural practice that can potentially reverse this trend by promoting soil health and increasing OC content.
7.2. Mechanisms of Intercropping in Enhancing Tropical Soil Organic Carbon Content
Intercropping can enhance OC content in tropical soils through several mechanisms. First, intercropping can increase the input of organic matter into the soil through the diverse and continuous supply of crop residues and root exudates from different crops grown together [97]. These crop residues and root exudates are rich in OC and serve as a source of energy for soil microorganisms, stimulating microbial activity and promoting the decomposition of organic matter, leading to higher OC content in the soil [122].
Second, intercropping can enhance the diversity and activity of soil microorganisms, including bacteria, fungi, and other microbes, which play a critical role in the decomposition of organic matter and the formation of stable soil OC pools [123]. The presence of multiple crops with different root exudates and residues in an intercropping system can create a more favorable environment for diverse microbial populations, leading to increased decomposition of organic matter and higher OC content in the soil [97].
Third, intercropping can improve nutrient cycling and nutrient use efficiency, which can influence the accumulation of OC in tropical soils. For example, legume intercropping, where leguminous crops are grown with non-leguminous crops, can fix atmospheric nitrogen through symbiotic nitrogen fixation with the help of nitrogen-fixing bacteria in their root nodules [124]. The fixed nitrogen can then be transferred to the associated non-leguminous crops, promoting their growth and reducing the need for synthetic nitrogen fertilizers. This can result in higher crop residues and root exudates, leading to increased OC input into the soil [124].
Furthermore, intercropping can also improve the physical properties of tropical soils, such as soil structure and water infiltration capacity, which can indirectly influence OC content. Intercropping systems with deep-rooted crops can help break up compacted soils and promote the formation of stable aggregates, which can protect organic matter from decomposition and promote its accumulation in the soil [27]. Additionally, intercropping can improve water-use efficiency by reducing soil erosion and enhancing water infiltration, leading to a more favorable soil moisture regime for organic matter decomposition and OC accumulation [122].
It is important to note that the magnitude of the increase in soil OC content through intercropping can vary depending on various factors, such as crop species, cropping patterns, management practices, and environmental conditions. For instance, legume intercropping has been found to have a greater impact on soil OC content compared to other types of intercropping due to the additional nitrogen fixation and higher input of organic matter [125]. Hence, intercropping has emerged as a promising agricultural practice that can increase OC content in tropical soils. The diverse crop residues and root exudates, enhanced microbial activity, improved nutrient cycling, and physical properties of soils promoted by intercropping can contribute to the accumulation of OC in the soil. However, it is important to consider site-specific factors and optimize management practices to maximize the benefits of intercropping for soil OC sequestration in tropical agricultural systems. Further research is needed to better understand the underlying mechanisms and long-term sustainability of intercropping practices in enhancing soil OC content in tropical soils.
8. AGROFORESTRY
8.1. Impact of Agroforestry Systems on Soil Organic Matter and Plant Biomass Production
Agroforestry systems, which involve the deliberate integration of trees with crops and/or livestock, have gained recognition as a sustainable land-use practice that can provide multiple benefits, including improving soil health and increasing OC content in tropical soils. Organic carbon content in soil is a critical indicator of soil fertility and plays a crucial role in supporting plant growth, water retention, nutrient cycling, and overall ecosystem sustainability. In this article, we will explore how agroforestry systems can contribute to increasing OC content in tropical soils, drawing upon relevant scientific literature.
One of the key mechanisms through which agroforestry systems enhance OC content in tropical soils is through increased biomass production and litterfall. Trees in agroforestry systems can sequester significant amounts of atmospheric carbon dioxide (CO2) through photosynthesis and allocate a substantial proportion of this carbon belowground as root exudates, root turnover, and litterfall, which adds to the organic matter pool in soil [73]. Litterfall, which consists of fallen leaves, branches, and other plant residues, contributes to the buildup of organic matter on the soil surface and its subsequent incorporation into the soil matrix, leading to increased OC content [126]. Agroforestry systems with tree species that have high leaf litter production rates, such as leguminous trees, can particularly enhance OC accumulation in soil due to their high-quality litter, which has a higher C-to-nutrient ratio and decomposes more slowly compared to lower-quality litter, thus increasing soil OC content over time (Nair et al., 2009).
Furthermore, agroforestry systems can promote the formation of stable soil organic matter through the incorporation of recalcitrant carbon compounds, such as lignin and cellulose, into the soil matrix. The presence of trees in agroforestry systems can increase the input of lignin-rich plant residues into the soil, which are relatively resistant to decomposition and can form stable complexes with soil minerals, protecting them from further decomposition [127]. This promotes the formation of long-term stable OC in the soil, contributing to the buildup of OC content over time. Agroforestry systems that include diverse tree species and promote a diverse microbial community in the soil can also enhance the formation of recalcitrant organic matter by influencing the composition and activity of soil microbial communities, which can selectively decompose different types of plant residues [128].
8.2. Impact of Agroforestry Systems on Soil Physical Properties, Nutrient Cycling, and Availability
Another important aspect of agroforestry systems that can contribute to increased OC content in tropical soils is the improvement of the soil's physical properties. Agroforestry systems with tree cover can protect the soil surface from direct sunlight, rainfall impact, and erosive forces, reducing soil erosion and promoting the formation of soil aggregates. These aggregates, composed of organic matter and mineral particles, are more stable and can protect OC from decomposition, leading to increased OC content in the soil [73]. Agroforestry systems with deep-rooted trees can also enhance soil structure by improving soil porosity, aeration, and water infiltration rates, which can facilitate the incorporation of organic matter into the soil matrix and increase organic C content [129].
Furthermore, agroforestry systems can contribute to increased OC content in tropical soils by promoting nutrient cycling and increasing nutrient availability. Trees in agroforestry systems can take up nutrients from deeper soil layers, where they may be less available to shallow-rooted crops, and recycle them through litterfall and root turnover, making them more accessible to other plants [73]. This nutrient cycling can enhance the productivity of crops and promote the incorporation of organic matter into the soil, leading to increased OC content. Moreover, leguminous trees commonly used in agroforestry systems can fix atmospheric nitrogen through symbiotic associations with nitrogen-fixing bacteria in their root nodules. This nitrogen fixation process can increase nitrogen availability in the soil, which can further promote plant growth and contribute to increased OC content in tropical soils [73].
Several studies have provided evidence of the positive impact of agroforestry systems on increasing OC content in tropical soils. For example, a study conducted in a coffee agroforestry system in Costa Rica found that the organic C content in the topsoil was significantly higher in agroforestry plots compared to adjacent conventional coffee monoculture plots, indicating that agroforestry promoted the accumulation of organic C in the soil [130]. Similarly, a study conducted in Bangladesh showed that agroforestry systems with different tree species had higher soil organic C content compared to adjacent monoculture systems, with the highest organic C content observed in agroforestry systems with leguminous trees [131].
Additionally, a meta-analysis of studies conducted in different tropical regions found that in agroforestry systems, more soil carbon sequestration occurs in agroforestry systems classified as silvopastoral and more above-ground carbon sequestration occurs in improved fallows. On average, carbon benefits are greater in agroforestry systems located in tropical climates when compared to agroforestry systems located in other climates [132]. These findings highlight the potential of agroforestry systems to increase OC content in tropical soils and contribute to soil health and sustainability.
It is important to note that the impact of agroforestry systems on OC content in tropical soils can vary depending on various factors, such as tree species, management practices, climate, and soil type. For instance, studies have shown that the type of tree species and their litter quality can significantly influence the rate of OC accumulation in soil, with leguminous trees generally having a greater impact due to their higher-quality litter and nitrogen-fixing abilities [133, 134]. In addition, management practices, such as pruning, mulching, and intercropping, can also affect the amount and quality of litterfall, nutrient cycling, and soil physical properties, which can, in turn, influence the OC content in soil [129, 135]. Climate and soil type can also play a role, as factors, such as temperature, precipitation, and soil texture, can influence the rate of organic matter decomposition, nutrient availability, and soil erosion, which can affect OC accumulation in soil [136].
Agroforestry systems have the potential to increase OC content in tropical soils through various mechanisms, including increased biomass production and litterfall, promotion of stable soil organic matter formation, improvement of soil physical properties, and enhanced nutrient cycling. However, the impact of agroforestry systems on OC content in tropical soils can be influenced by multiple factors and may vary depending on specific site conditions and management practices. Further research and long-term monitoring are needed to better understand the dynamics of organic C accumulation in agroforestry systems and optimize management practices for sustainable soil health and productivity in tropical agroecosystems.
9. BIOCHAR
Biochar, a type of charcoal produced from biomass pyrolysis, has gained considerable attention in recent years as a potential tool for soil carbon sequestration and soil fertility improvement. Tropical soils, which are often characterized by low OC content and nutrient deficiencies, can greatly benefit from the application of biochar. In this article, we will explore the mechanisms by which biochar can increase OC content in tropical soils, supported by relevant scientific references.
9.1. Organic Carbon Sequestration
One of the primary mechanisms through which biochar increases OC content in tropical soils is through its recalcitrance or stability. Biochar is resistant to decomposition and can persist in soil for hundreds to thousands of years, depending on its production conditions and feedstock properties [137, 138]. This means that when biochar is applied to tropical soils, it can effectively sequester OC, preventing its decomposition and loss to the atmosphere as carbon dioxide (CO2). Several studies have demonstrated that biochar can significantly increase the OC content of tropical soils. For example, a study conducted in Bangladesh by Rahman et al. [139] showed that biochar made from sugarcane bagasse increased soil OC content from 42% to 58%. Moreover, biochar can also enhance the stabilization of native soil organic matter (SOM) in tropical soils. SOM is the organic fraction of soil that plays a crucial role in soil fertility, water retention, and carbon cycling.
9.2. Biochar-enhanced SOM, Microbial Activity, and Biochemical Properties of Tropical Soils
Biochar can increase the sorption of SOM onto its porous surface, reducing its availability for microbial decomposition [140, 141]. This can result in the build-up of SOM in the soil, leading to an increase in OC content. For example, a study conducted in the Amazonian Dark Earths, a type of fertile anthropogenic soil found in tropical regions, showed that biochar application increased the concentration of recalcitrant SOM. Carbon sequestration was 3.4 times higher in the Terra Preta than in adjacent soils [142]. Biochar can also promote the formation of stable soil aggregates, which are important for soil structure and stability and can protect OC from decomposition. The porous structure of biochar can provide sites for microbial colonization, which can stimulate the formation of soil aggregates through microbial activities and interactions with other soil particles [137, 143].
Furthermore, biochar can enhance microbial activity and diversity in tropical soils, which can contribute to the accumulation of OC. Biochar provides a favorable habitat for microbial communities, including bacteria, fungi, and archaea, due to its high surface area, porous structure, and nutrient content [137]. These microorganisms can decompose complex organic compounds in biochar, releasing nutrients and metabolites that can stimulate plant growth and enhance the cycling of OC in the soil. In addition, biochar can stimulate the growth of beneficial microbial communities, such as mycorrhizal fungi, which can facilitate plant nutrient uptake and enhance soil OC sequestration [137]. This can result in increased plant biomass production and subsequent inputs of plant residues into the soil, leading to higher OC content. For example, a study conducted in Nigeria by Adekiya et al. [144] showed that biochar application increased the abundance of beneficial microbes, which contributed to increased plant growth and higher OC content in the soil.
Furthermore, biochar can also influence the biochemical properties of tropical soils, which can affect OC dynamics. Biochar has been shown to alter soil pH, cation exchange capacity (CEC), and nutrient availability, which can impact soil organic matter decomposition rates and stabilization [144, 145]. For example, some studies have reported that biochar can increase soil pH, which can slow down microbial decomposition of organic matter and promote its accumulation in the soil [146, 147]. Additionally, biochar can increase CEC, which can enhance the retention of organic matter and nutrients in the soil, thereby promoting the formation of stable soil organic matter complexes [137, 143]. These changes in soil biochemical properties can contribute to the increase in OC content in tropical soils.
In addition to the above mechanisms, the effect of biochar on the OC content of tropical soils can also depend on various factors, such as biochar properties (e.g., feedstock, production temperature, and duration), application rate, soil type, and environmental conditions (e.g., temperature, moisture, and vegetation) [137]. Different types of biochar can have different effects on organic C dynamics in tropical soils. For instance, biochars produced at higher temperatures or from feedstocks with higher lignin content tend to be more recalcitrant and stable, leading to higher OC sequestration potential [137, 138]. The application rate of biochar can also influence the organic C content, as higher application rates can lead to greater inputs of biochar-derived OC into the soil, resulting in higher OC accumulation [144]. Moreover, soil type and environmental conditions can also affect the interactions between biochar and tropical soils, as different soils have different properties and microbial communities that can influence biochar's effectiveness in increasing OC content [137].
Therefore, biochar has the potential to increase OC content in tropical soils through various mechanisms, including its recalcitrance, promotion of SOM stabilization, formation of stable soil aggregates, stimulation of microbial activity and diversity, and alteration of soil biochemical properties. However, the effectiveness of biochar in increasing OC content can be influenced by biochar properties, application rate, soil type, and environmental conditions. Further research is needed to better understand the interactions between biochar and tropical soils and optimize biochar application strategies for sustainable soil management and climate change mitigation.
CONCLUSION
In conclusion, increasing the OC content of tropical soils is a multifaceted approach that requires the implementation of various conservation practices. Conservation tillage practices can reduce soil disturbance and respiration from soils, while crop residue retention can increase organic matter inputs and soil nutrients, promote soil water retention, and reduce soil erosion. Cover crops and crop rotation can prevent nutrient losses and improve soil organic carbon through increased vegetation biomass. Fertilizer application, manure and compost application, intercropping, agroforestry, and biochar are also important strategies to enhance soil organic carbon. By adopting these practices, farmers and land managers can improve soil health, increase crop productivity, and mitigate climate change by sequestering more carbon in tropical soils. However, further research and monitoring are needed to understand the long-term impacts of these practices and optimize their effectiveness in different tropical regions. Implementing these strategies in a holistic and integrated manner can contribute to sustainable agricultural production, climate change mitigation, and resilience of tropical soils for future generations.
LIST OF ABBREVIATIONS
SOC | = Soil Organic Carbon |
OC | = Organic Carbon |
OM | = Organic Matter |
OC | = Organic Carbon |
CONSENT FOR PUBLICATION
Not applicable.
FUNDING
None.
CONFLICT OF INTEREST
The authors declare no conflict of interest, financial or otherwise.
ACKNOWLEDGEMENTS
Declared none.